Stereotaxic Impactor
The stereotaxic impactor is designed to induce traumatic brain injury (TBI) through controlled cortical impact modeling. This advanced equipment allows researchers to create highly reproducible neurotrauma models, ensuring precise impact direction and injury location.Ā
It provides researchers with full control over the biomechanical parameters of brain injury, including velocity, depth, dwell time, and the force applied by the tip. ConductScienceās Stereotaxic Impactor also allows for customization of the tip size, geometry, and position to accommodate different species. Together, these features enable researchers to address multiple histopathological and functional questions related to brain injury, making it an indispensable tool in neurotrauma research.
Applications of the Stereotaxic Apparatus
Stereotaxic procedures are frequently used to eliminate, enhance, or modify the function of certain brain regions as part of neurological research, pharmacological evaluation or central nervous system (CNS) disease-related experiments. [1]
The apparatus is a versatile and indispensable tool in both preclinical and clinical research settings. Its applications span a wide range of fields within neuroscience and beyond, providing precise localization and manipulation of specific brain regions. Some key applications include:
Neuroscience Research:
- Brain Mapping: Researchers use the stereotaxic apparatus to map brain functions by precisely targeting and manipulating specific areas. This helps in understanding the roles of different brain regions in behavior, cognition, and sensory processing. [2]
- Neurotransmitter Studies: The apparatus enables the accurate injection of chemicals, allowing the study of neurotransmitter systems. Techniques such as microdialysis can be employed to measure neurotransmitter release and uptake in specific brain regions. [3]
- Electrophysiology: It facilitates the placement of electrodes to record electrical activity from neurons, providing insights into the neural basis of behavior and disease. [4]
Ā
Pharmacological Research:
- Drug Delivery: Precisely delivers pharmacological agents directly into targeted brain areas, allowing for the study of drug effects on specific neural circuits. [5]
- Gene Therapy: The apparatus can deliver viral vectors carrying genetic material to specific brain regions, aiding in gene therapy research for neurological disorders. [6]
Ā
Behavioral Studies:
- Lesion Studies: By creating controlled lesions in specific brain areas, researchers can study the resulting behavioral changes, contributing to our understanding of brain function and dysfunction. [7]
- Implantation of Devices: Chronic implantation of devices such as cannulas or optical fibers allows for long-term manipulation and observation of neural activity during behavioral experiments. [8]
Ā
Advanced Neuroscience Techniques:
- Optogenetics: This technique involves the insertion of light-sensitive proteins into neurons, which can then be controlled with light. The stereotaxic apparatus ensures the precise placement of optical fibers for light delivery. [9]
- Fiber Photometry: It allows real-time monitoring of neural activity by measuring fluorescent signals from genetically encoded indicators. Precise placement of optical fibers is crucial for accurate data collection. [10]
- Two-Photon Imaging: For high-resolution imaging of neuronal activity, the apparatus is used to stabilize and target the imaging equipment to specific brain regions. [11]
Ā
Clinical Applications:
- Deep Brain Stimulation (DBS): In the clinical setting, the stereotaxic apparatus is essential for the precise placement of electrodes in the treatment of conditions like Parkinsonās disease, essential tremor, and dystonia. [12]
- Biopsies: Stereotaxic apparatus allows for the accurate collection of brain tissue samples for diagnostic purposes, minimizing damage to surrounding healthy tissue. [13]
Ā
Developmental Studies:
Embryonic and Neonatal Research: The apparatus can be adapted for use with very small animal subjects, such as embryos or neonates, facilitating studies on brain development and the effects of early-life interventions. [14]
Traumatic Brain Injury (TBI) Induction
Traumatic brain injury is one of the leading causes of morbidity and mortality among young people around the world. [15]Ā
Animal models are commonly used to study brain trauma and test new treatments. The Controlled Cortical Impact (CCI) model is the most popular and accepted method for researching the physical, mental, and behavioral problems that result from brain injury. CCI is favored because it allows precise control over how the brain tissue is damaged. [16]
Hereās a step by step guide for TBI induction:
- Set Up the Stereotaxic Instrument: Prepare all necessary materials, ensuring the area and instruments are thoroughly cleaned and sterilized. [17]
- Shave the Fur: Use an electric razor to shave the fur from the ears to just between the eyes, moving in different directions to clean the area effectively. Apply povidone-iodine to the shaved area, avoiding the eyes. [17]
- Mount the Animal: Secure the animal onto the stereotaxic apparatus by inserting the ear bars into the ear canal and tightening them. Ensure the head is immobile and level with a ruler. Confirm the 90° angle between the ruler and the animalās scalp using the stereotaxic instrument. [17]
- Make the Incision: Using a sterile scalpel, make an anterior/posterior incision on the scalp from the lambda (a point at the back of the skull) to between the eyes. Pinch the skin with sterilized hemostats to keep the incision open and dry the exposed skull surface with sterile cotton swabs. [17]
- Position the Guide Cannula: Mount the guide cannula and locate bregma on the skull. Record the anterior/posterior and lateral coordinates, then find the correct coordinates for probe placement using a stereotaxic atlas. Adjust the guide cannula to the correct coordinates and record the ventral coordinate. Mark the drilling spot on the skull with a sterile pencil. [17]
- Drill the Hole: Sterilize the drill bit and carefully drill a hole at the marked location until you penetrate the skull. Ensure the guide cannula can pass through the hole without touching the sides. Use a sterile needle to puncture the meninges for unobstructed cannula insertion. [17]
- Prepare for Skull Screws: Using a hand drill, create six holes for skull screws: two anterior, two lateral, and two posterior to the cannula hole. Sterilize the screws and anchor them tightly onto the skull. [17]
- Insert the Guide Cannula: Clean the guide cannula with ethanol and saline, then mount and lower it to the proper ventral coordinate, ensuring a perfect vertical insertion without touching the sides. [17]
- Secure with Dental Cement: Place an anchor screw medially behind the posterior skull screws. Mix a thin batch of liquid dental cement to cover the guide cannula, screws, and skull. Apply a thicker batch to secure the area, ensuring smoothness to prevent skin irritation. [17]
- Finish the Cement Application: As the cement thickens but before it solidifies, separate the skin from the cement cap and mold it to ensure smoothness. [17]
- Allow Cement to Dry: Let the dental cement dry completely before removing the animal from the apparatus. Remove the hemostats and apply bacitracin around the cement cap. [17]
- Fit the monoinjector to the stereotaxic frame: Lower the needle into the hole to the target DV coordinate and inject. Leave the injection needle in place for at least 30 sec after completion of the injection and before removal of the needle. [18]
- Remove the monoinjector and seal the hole with bone wax. Suture the incision and remove the animal from the stereotaxic frame. [18]
- Post-Procedure Injections: Administer 0.25 ml of penicillin intramuscularly, followed by 1 ml of saline subcutaneously. [17]
- Monitor Recovery: Place the animal in its cage and monitor it until consciousness is regained. Return it to its room for recovery. [17]
- Post-Op Monitoring: Check animals daily for signs of infection, pain, or distress until the end of the experiment. Look for low movement, distress vocalization, hunched posture, diarrhea, swelling, discharge, and lack of feeding/drinking. Administer buprenorphine for pain and antibiotics for infections as needed. Euthanize the animal if symptoms persist despite treatment. [17]
Conclusion
The stereotaxic apparatus is an essential tool in neuroscience and biomedical research. It is crucial for brain injury research as it provides precise and controlled induction of TBI.
This tool allows researchers to replicate brain injuries with high accuracy in terms of impact location and severity, ensuring consistency across experiments. By using the stereotaxic impactor, scientists can study the physiological, histological, and behavioral effects of TBI in a reproducible manner.Ā
This precision is essential for understanding the mechanisms of brain injuries and for developing effective treatments and therapies.
References
- Yang, P., Wang, Z., Zhang, Z., Liu, D., Manolios, E. N., Chen, C., Yan, X., Zuo, W., & Chen, N. (2018). The extended application of The Rat Brain in Stereotaxic Coordinates in rats of various body weight. Journal of neuroscience methods, 307, 60ā69. https://doi.org/10.1016/j.jneumeth.2018.06.026
- Paxinos, G., & Watson, C. (2007). The rat brain in stereotaxic coordinates: Hard cover edition. Elsevier.
- Benveniste, H., & Hansen, A. J. (1990). Microdialysis: Theory and application. Progress in Neurobiology, 35(3), 195-215.
- BuzsƔki, G. (2004). Large-scale recording of neuronal ensembles. Nature Neuroscience, 7(5), 446-451.
- Paxinos, G., & Watson, C. (1998). The rat brain in stereotaxic coordinates (4th ed.). Academic Press.
- Davidson, B. L., & Breakefield, X. O. (2003). Viral vectors for gene delivery to the nervous system. Nature Reviews Neuroscience, 4(5), 353-364.
- Kandel, E. R., Schwartz, J. H., & Jessell, T. M. (2000). Principles of Neural Science (4th ed.). McGraw-Hill.
- Paxinos, G., & Watson, C. (2005). The mouse brain in stereotaxic coordinates. Elsevier.
- Deisseroth, K. (2011). Optogenetics. Nature Methods, 8(1), 26-29.
- Cui, G., Jun, S. B., Jin, X., Luo, G., Pham, M. D., Lovinger, D. M., & Costa, R. M. (2013). Deep brain optical measurements of cell typeāspecific neural activity in behaving mice. Nature Protocols, 8(8), 1609-1621.
- Helmchen, F., & Denk, W. (2005). Deep tissue two-photon microscopy. Nature Methods, 2(12), 932-940.
- Benabid, A. L., Pollak, P., Gao, D., Hoffmann, D., Limousin, P., Gay, E., & Perret, J. (1996). Chronic electrical stimulation of the ventralis intermedius nucleus of the thalamus as a treatment of movement disorders. Journal of Neurosurgery, 84(2), 203-214.
- Apuzzo, M. L., & Heiden, J. S. (1984). The use of computed tomography in stereotactic surgery. Acta Neurochirurgica, 70(1), 21-31.
- Paxinos, G., & Franklin, K. B. J. (2001). The mouse brain in stereotaxic coordinates (2nd ed.). Academic Press.
- Mansvelder, F. J., Bossers, S. M., Loer, S. A., Bloemers, F. W., Van Lieshout, E. M. M., Den Hartog, D., Hoogerwerf, N., van der Naalt, J., Absalom, A. R., Peerdeman, S. M., Bulte, C. S. E., Schwarte, L. A., Schober, P., & BRAIN-PROTECT collaborators (2024). Etomidate versus Ketamine as Prehospital Induction Agent in Patients with Suspected Severe Traumatic Brain Injury. Anesthesiology, 140(4), 742ā751. https://doi.org/10.1097/ALN.0000000000004894
- Osier, N. D., & Dixon, C. E. (2016). The controlled cortical impact model: Applications, considerations for researchers, and future directions. Frontiers in Neurology, 7, 134. https://doi.org/10.3389/fneur.2016.00134
- Geiger BM, Frank LE, Caldera-Siu AD, Pothos EN. Survivable stereotaxic surgery in rodents. J Vis Exp. 2008 Oct 6;(20):880. doi: 10.3791/880. PMID: 19078946; PMCID: PMC3233859.
- Athos, J., & Storm, D. R. (2001). High precision stereotaxic surgery in mice. Current protocols in neuroscience, Appendix 4. https://doi.org/10.1002/0471142301.nsa04as14
See more of Our Posts
stereotaxic on resources
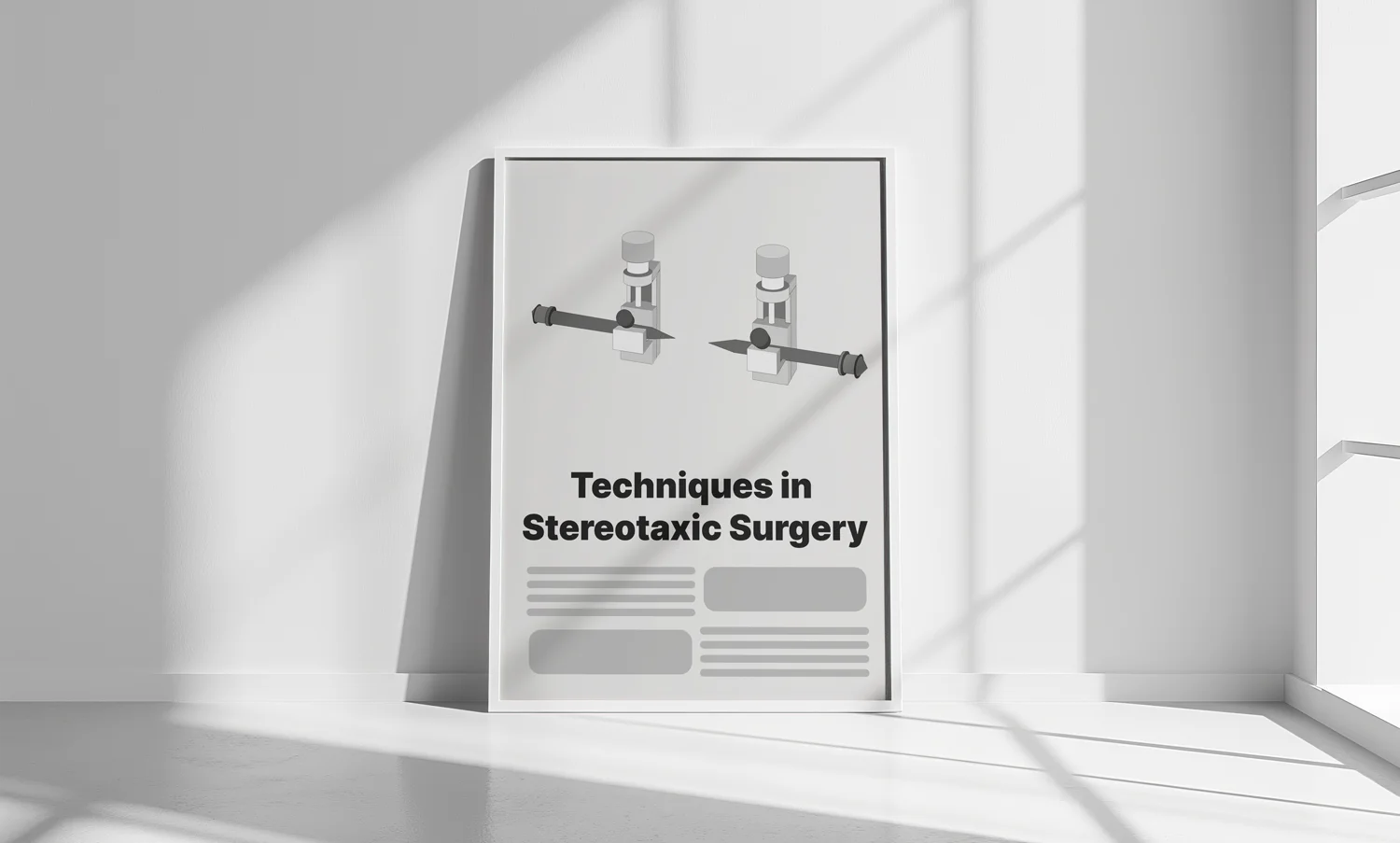
Techniques in Stereotaxic Surgery
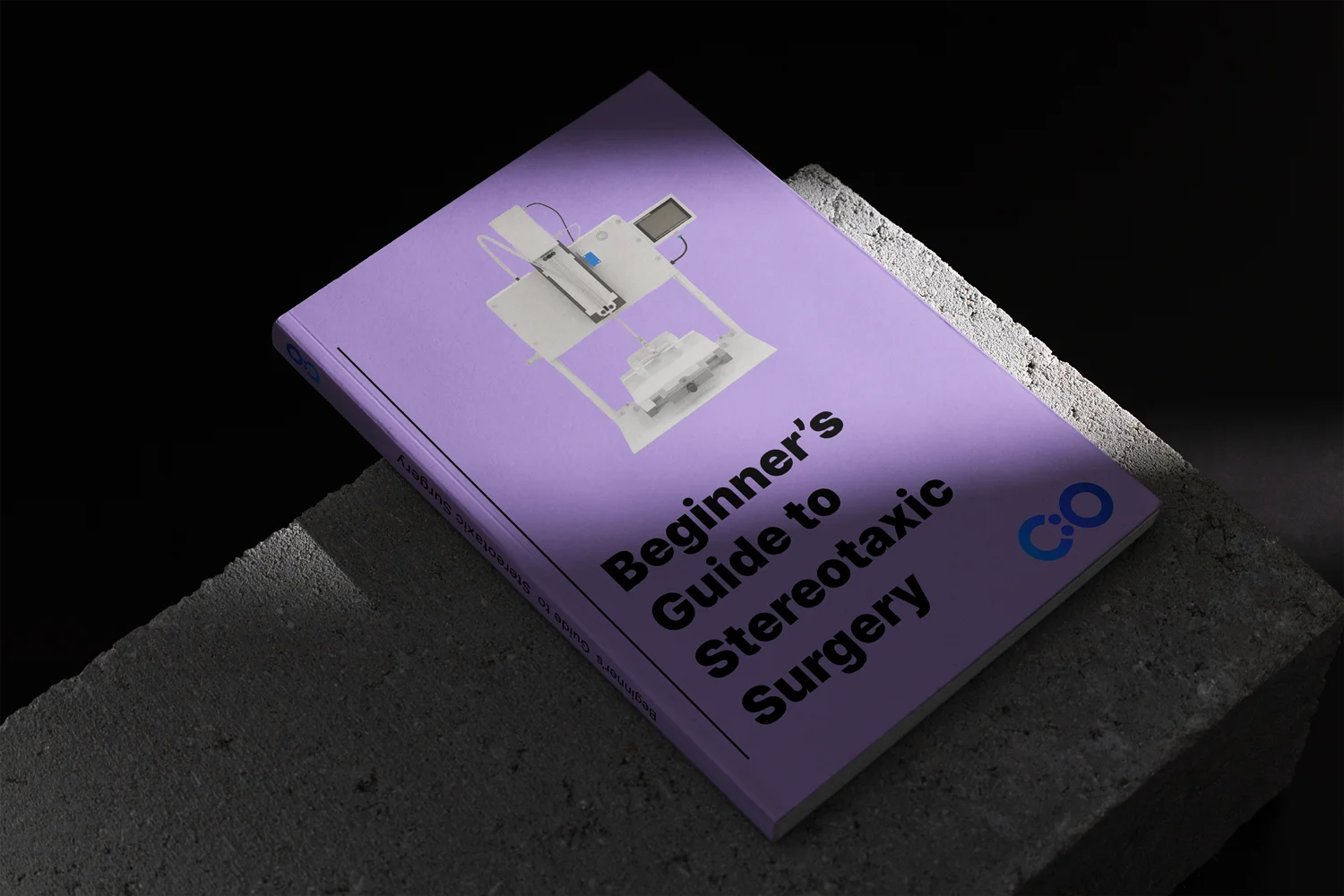
Beginner’s Guide to Stereotaxic Surgery
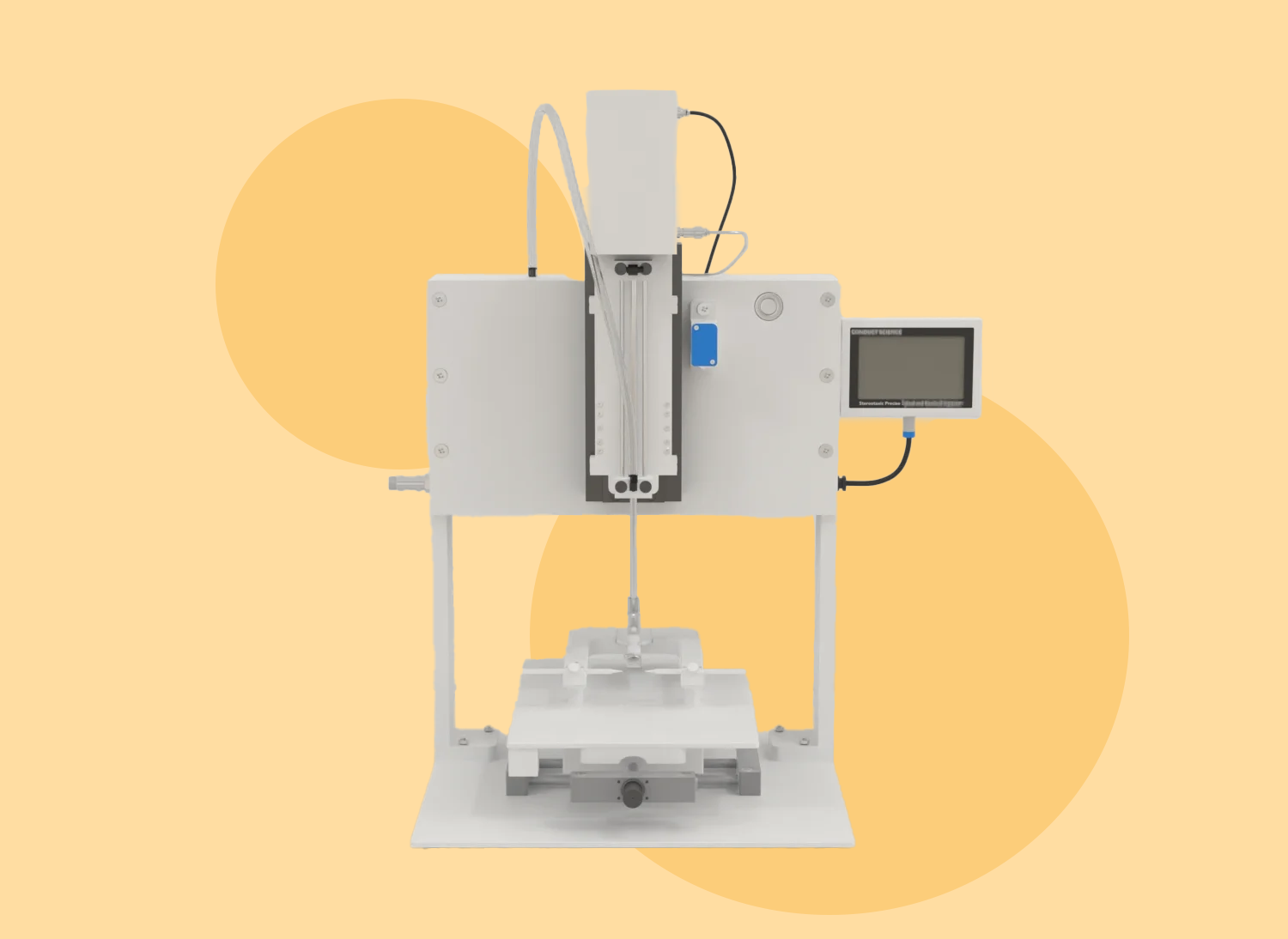
Stereotaxic Impactor for Traumatic Brain Injury (TBI) Induction
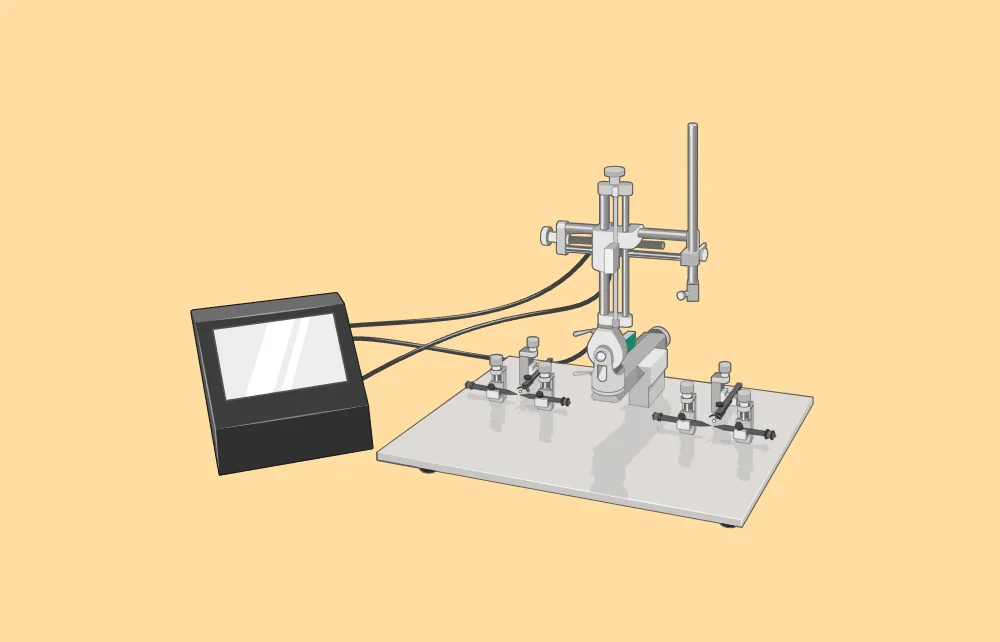
Applications of the Stereotaxic Apparatus
All Resources
Never miss our posts!
Author:

Louise Corscadden, PhD
Dr Louise Corscadden acts as Conduct Science’s Director of Science and Development and Academic Technology Transfer. Her background is in genetics, microbiology, neuroscience, and climate chemistry.