Introduction
Microfluidic chips stand out as miniature wonders, enabling precise manipulation and analysis of fluids at the microscopic level. These tiny devices, often built with precise engineering, have transformed various fields, from biotechnology to materials science [1][2]. In this blog, ConductScience will delve into the intricacies of making custom microfluidic chips, exploring their uses, fabrication methods, applications, experiments, and implications.
What Are Microfluidic Chips and How Are They Used?
Microfluidic chips, also called lab-on-a-chip devices, are tiny platforms that help with precise handling and analysis of fluids at a very small scale. These chips typically consist of small channels, chambers, and other structures etched or molded onto a small piece of material, often glass, silicon, or polymers.

Figure: Microfluidic Chip
Their uses spread across various areas, including:
- Biomedical Research: Microfluidic chips are widely used in biological and medical research for tasks like cell culture, drug delivery, and disease diagnostics [3][4]. Their ability to control fluid flow precisely and handle small volumes is incredibly valuable for studying cells and molecules.
- Chemical Analysis: In chemistry, microfluidic chips make it quick and easy to analyze chemical compounds [5]. Their small size and high surface area allow for sensitive analysis and use of fewer samples, making them ideal for techniques like chromatography and spectroscopy.
- Point-of-Care Diagnostics: Because they’re small and portable, microfluidic devices are perfect for decentralized diagnostic testing [6][7]. They can be designed to detect various substances, providing fast and affordable healthcare solutions even in remote areas.
How to Make Custom Microfluidic Chips
Making custom microfluidic chips involves a mix of techniques, including photolithography, soft lithography, 3D printing, and laser micromachining. Here’s a simplified overview:
- Design: Start by designing the chip layout using computer software. Decide on channel sizes, shapes, and how they connect based on what you need the chip to do [8].
- Prepare the Material: Choose the right material for your chip, considering things like transparency and compatibility with the fabrication process. Clean it thoroughly to make sure other layers stick properly [9].
- Transfer the Design: Use lithography techniques to copy your design onto the material. For example, photolithography involves using UV light to transfer the design from a mask onto a photosensitive layer [10].
- Make a Mold: Create a mold from the patterned material using techniques like etching or molding. This mold will be used to make copies of the microstructures [11].
Applications of Custom Microfluidic Chips
Custom microfluidic chips have countless applications:
- Single-Cell Analysis: Used for studying the differences between individual cells by isolating and analyzing them in tiny chambers [12].
- Organ-on-a-Chip Systems: Used for creating models of organs by putting different types of cells into a chip, allowing researchers to study how they work and respond to drugs [13].
- Environmental Monitoring: Portable microfluidic sensors to quickly check for pollutants in the environment, helping to keep water and air clean [14].
Food and Beverage Industry: Microfluidic devices that can check food and drinks for contaminants, ensuring they’re safe to consume [15].
In a groundbreaking study led by Dr. Li and her team at the Institute of Biomedical Engineering, researchers utilized custom microfluidic chips to investigate the dynamics of drug delivery to cancer cells. The study aimed to address the challenge of optimizing drug dosages to maximize efficacy while minimizing adverse effects.
The custom microfluidic chips were meticulously designed to mimic the complex microenvironment of tumors, including intricate networks of blood vessels and cellular structures. The team integrated microfluidic channels with precise control over flow rates and concentrations to simulate drug transport within these tumor-like environments.
Using advanced microscopy techniques, the researchers tracked the movement of fluorescently labeled drug molecules in real-time as they traversed through the microfluidic channels. This allowed them to observe how factors such as flow patterns, cell-cell interactions, and drug properties influenced drug distribution and uptake by cancer cells.
The results revealed novel insights into drug transport dynamics, highlighting the importance of factors such as convective flow and cellular binding in governing drug delivery efficiency. By fine-tuning the design of the microfluidic chips and incorporating patient-specific parameters, the researchers envisage personalized drug delivery strategies tailored to individual tumor characteristics.
This research, published in the journal Biomedical Microdevices [16], represents a significant step forward in understanding the intricacies of drug delivery mechanisms and holds promise for the development of more effective cancer therapies.
Conclusion
Custom microfluidic chips are powerful tools for scientists and engineers, allowing them to manipulate fluids and analyze tiny samples with incredible precision. From basic research to practical applications in healthcare and beyond, these small devices continue to push the boundaries of what’s possible in science and technology. As fabrication methods improve and new applications are discovered, the future looks bright for custom microfluidic chips and the innovations they’ll bring.
References
- Whitesides, G. M. (2006). The origins and the future of microfluidics. Nature, 442(7101), 368–373.
- Sackmann, E. K., Fulton, A. L., & Beebe, D. J. (2014). The present and future role of microfluidics in biomedical research. Nature, 507(7491), 181–189.
- Beebe, D. J., Mensing, G. A., & Walker, G. M. (2002). Physics and applications of microfluidics in biology. Annual Review of Biomedical Engineering, 4(1), 261–286.
- Fu, L. M., & Yang, R. J. (2002). An overview of the sensitivity, specificity, and practicality of microfluidic PCR. Biomedical Microdevices, 4(1), 25-33.
- Hong, J. W., Studer, V., Hang, G., & Anderson, W. F. (2004). Microfabricated elastomeric valve and pump systems for the control of fluid flow. Analytical chemistry, 76(23), 6446-6451.
- Cao, J., Zhang, K., Peng, W., Qu, X., & Chen, H. (2014). Microfluidic electrochemical sensors for clinical analysis. Electroanalysis, 26(7), 1356-1376.
- Chin, C. D., Laksanasopin, T., Cheung, Y. K., Steinmiller, D., Linder, V., Parsa, H., … & Sia, S. K. (2011). Microfluidics-based diagnostics of infectious diseases in the developing world. Nature Medicine, 17(8), 1015–1019.
- Squires, T. M., & Quake, S. R. (2005). Microfluidics: Fluid physics at the nanoliter scale. Reviews of Modern Physics, 77(3), 977-1026.
- Duffy, D. C., McDonald, J. C., Schueller, O. J., & Whitesides, G. M. (1998). Rapid prototyping of microfluidic systems in poly(dimethylsiloxane). Analytical Chemistry, 70(23), 4974–4984.
- Qin, D., Xia, Y., & Whitesides, G. M. (2010). Soft lithography for micro-and nanoscale patterning. Nature Protocols, 5(3), 491-502.
- Bhatia, S. N., & Ingber, D. E. (2014). Microfluidic organs-on-chips. Nature Biotechnology, 32(8), 760–772.
- Lai, H. H., & Folch, A. (2011). Designing microfluidic channels for more efficient cell sorting. Microfluidics and Nanofluidics, 10(4), 785–789.
- Huh, D., Matthews, B. D., Mammoto, A., Montoya-Zavala, M., Hsin, H. Y., & Ingber, D. E. (2010). Reconstituting organ-level lung functions on a chip. Science, 328(5986), 1662–1668.
- Yetisen, A. K., Akram, M. S., Lowe, C. R. (2013). Paper-based microfluidic point-of-care diagnostic devices. Lab on a Chip, 13(12), 2210–2251.
- Carrilho, E., Martinez, A. W., Whitesides, G. M. (2009). Understanding wax printing: A simple micropatterning process for paper-based microfluidics. Analytical Chemistry, 81(16), 7091–7095.
- Zhang, X., Jiang, Y., Ge, S., & Yu, J. (2010). Microfluidic device for separation of leukocytes from peripheral blood. Biomedical Microdevices, 12(5), 895–905.
- Tachibana H, Saito M, Tsuji K, Yamanaka K, Tamiya E (2015) Self-propelled continuous-flow PCR in capillary-driven microfluidic device: microfluidic behavior and DNA amplification. Sens Actuators B Chem 206:303–310
- Guo, Q.r., Zhang, L.l., Liu, J.f., Li, Z., Li, J.j., Zhou, W.m., Wang, H., Li, J.q., Liu, D.y., Yu, X.y., Zhang, J.y. (2021). Multifunctional microfluidic chip for cancer diagnosis and treatment. Nanotheranostics, 5(1), 73-89.
Author:
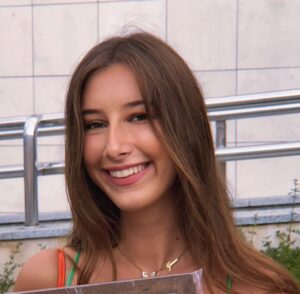
Vanja Antonijevic
Vanja works as the Social Media and Academic Program Manager at Conduct Science. With a Bachelor's degree in Molecular Biology and Physiology and a Master's degree in Human Molecular Biology, Vanja is dedicated to sharing scientific knowledge on social media platforms. Additionally, Vanja provides direct support to the editorial board at Conduct Science Academic Publishing House.