Introduction
Microfluidic chips are only one piece of the puzzle in an experimental setup. To actually use a chip, you need to connect it with the outside world – introducing samples, driving fluids, and collecting outputs – while maintaining a clean, controlled environment. In this article, we provide a practical guide to integrating microfluidic chips into your lab workflow. We will cover how to interface chips with pumps and tubing, strategies to avoid common pitfalls like bubbles and sample loss, tips for efficient on-chip loading and imaging, and advice on maintaining and reusing chips.
Explore our microfluidic chip for sale
Fluidic Interfacing: Connecting Chips, Tubing, and Pumps
One of the first challenges is connecting macroscopic equipment (like pumps or syringes) to the microscopic channels of your chip. This typically involves some combination of tubing and connectors/fittings:
- Tubing: Microfluidic setups commonly use small-diameter tubing (e.g., 1/16 inch OD ~1.6 mm, or even 360 µm OD capillaries) to deliver fluids to and from the chip. The tubing material (Teflon PTFE, Tygon PVC, PEEK, etc.) should be chosen based on chemical compatibility and desired flexibility. Soft tubing (Tygon, silicone) is easy to handle and seal, often used with peristaltic pumps or syringe pumps (How to Connect your Tubing to a ChipShop Microfluidic Chip?). Rigid tubing (PTFE, PEEK) is inert and can handle high pressure but is less forgiving in connection (it doesn’t stretch to seal over ports) (How to Connect your Tubing to a ChipShop Microfluidic Chip?).
- Connectors and Fittings: These are used to join tubing to the chip’s inlet/outlet ports in a leak-tight manner. There are two major categories: Luer connections and threaded fittings (Microfluidics: How to Choose the Good Fittings & Connectors?).
- Luer connectors are the same tapered fittings used in medical syringes. Many commercial microfluidic chips (e.g., from ChipShop or others) come with molded Luer stubs at the inlet/outlet (How to Connect your Tubing to a ChipShop Microfluidic Chip?). You can simply press on a piece of soft tubing by hand (“press-fit”) onto the Luer until it’s snug (How to Connect your Tubing to a ChipShop Microfluidic Chip?). Luer connections are quick and user-friendly, ideal for low to medium pressures. They do, however, introduce a bit of dead volume and aren’t great for very high pressure (they might pop off if pressure spikes).
- Threaded fittings use a screw and ferrule mechanism (like in HPLC systems) to secure tubing (Microfluidics: How to Choose the Good Fittings & Connectors?). Common thread standards in microfluidics are 1/4-28 UNF or M6. These require the chip to have a threaded port or a receptor (often a plastic or metal port glued to the chip). The tubing is inserted through a nut and ferrule; as you tighten the nut, the ferrule compresses the tubing against the port, making a tight seal (Microfluidics: How to Choose the Good Fittings & Connectors?). Threaded fittings can handle high pressures (hundreds of psi, even >100 bar for PEEK fittings) (Microfluidics: How to Choose the Good Fittings & Connectors?) and are reliable for long-term setups. The downside is they usually require rigid tubing (since soft tubing will collapse under the ferrule compression) (Microfluidics: How to Choose the Good Fittings & Connectors?), or you need an adapter piece.
- There are also adapters between these types: e.g., a Luer-to-threaded adapter, or barbed fittings to connect soft tubing to a threaded port.
- For PDMS chips made in-house, often you don’t have a fancy molded port. A common method is to punch a hole and insert a small metal tube or a pipette tip. For example, a blunt hypodermic needle of diameter ~0.8 mm can be inserted into a PDMS port hole; then tubing can be slid over that needle as a barb. Alternatively, quick connect kits (like small barbed connectors) can be glued into PDMS. Ensuring a good seal is key – leaks at the interface cause unstable flows and can contaminate your setup.
- Pumps and Pressure Sources: You can drive fluid flow either by pushing liquid with a pump (syringe pump, peristaltic pump) or by pressurizing the fluid reservoirs (using compressed gas to push liquid into the chip). Syringe pumps are straightforward – they infuse or withdraw at a set flow rate. They are great for steady, low-pressure flow but can introduce pulsation at very low rates and have limited responsiveness (it’s hard to quickly change flow on a syringe pump due to inertia). Peristaltic pumps can cause pulsatile flow (because of the roller mechanism) unless you use a pulse dampener. Pressure controllers (like those from Fluigent or Elveflow) apply controlled air pressure on a liquid reservoir to drive flow; these offer very smooth and fast-responsive flow control, especially for complex multi-channel experiments. The choice of pump depends on what you have and the flow stability needed. For many basic uses, a syringe pump is fine. For more advanced flow control (like creating droplets or dynamic flow profiles), a pressure-based system is often preferred.
When connecting it all together, keep in mind:
- Minimize Dead Volume: Use the shortest tubing lengths possible and the smallest internal diameter that still meets your flow needs. Excess tubing volume means more sample wasted (it stays in the tube) and longer response times when you change conditions. For instance, 100 µL of dead volume in tubing might dilute or delay a reagent plug significantly. If you use connectors, choose those with small internal cavities (some Luer connectors have a larger internal chamber – alternatives like low-dead-volume connectors are available (Microfluidic design: Minimizing Internal Volumes – Translume)).
- Secure Connections: It sounds obvious, but ensure all connections are tight before starting an experiment. A loose fitting can draw in air or leak liquid. If using push-on connections, sometimes a small clamp or a bit of glue can secure it (but glue can be messy if you need to disconnect later).
- Alignment and Strain Relief: If your chip is small and light (like a thin PDMS/glass device), even the tubing can exert enough force to move it or stress it. It’s good to mount the chip on a holder or tape it down, and secure tubing so that it doesn’t pull on the chip. In high-density setups, use a fixture that holds connectors in place (some commercial manifolds align multiple connections to a chip in one piece, for example Dolomite’s linear connector for multiple channels ([PDF] multiflux-2 linear connectors – user instructions – Dolomite Microfluidics)).
By carefully selecting connectors and pumps, you’ll create a robust interface between your macro instruments and the micro world on the chip. A well-planned interfacing setup means you can swap chips in and out without re-plumbing everything each time, and you can trust that flow rates and pressures you set are actually what the chip experiences.
Preventing Air Bubbles, Contamination, and Sample Loss
When working at the microscale, seemingly minor issues like a tiny air bubble or a small reagent loss can ruin an experiment. Here’s how to mitigate these problems:
Air Bubbles: Bubbles are the bane of microfluidics. A single air bubble can block a channel, disrupt flow, create dead zones, or introduce severe errors (e.g., in cell culture chips, bubbles can kill cells by exposing them to air). Bubbles arise from various sources – dissolved gases coming out of solution, trapped air in connectors or dead-ends, leaks sucking air in, or outgassing from PDMS walls.
- Degassing fluids: Use degassed solvents and samples whenever possible. You can degas by vacuuming the liquid (placing it in a vacuum chamber for a few minutes) or by sonication under vacuum. Removing dissolved air greatly reduces bubble formation during flow (Addressing Air Bubble Issues in Microfluidic Systems – Fluigent).
- Prime and purge: Before running your actual experiment, flush the chip and tubing with a compatible liquid (e.g., DI water or buffer) to push out any air. Fill syringes carefully (avoid drawing up air). When connecting tubing, fill it with liquid first – for instance, back-fill a syringe and tubing with fluid so no air is inside, then connect to the chip port while a little fluid is spilling out (ensuring an air-free connection).
- Avoid sudden pressure drops: If using a pressure-based pump, don’t rapidly release pressure as that can cause dissolved gas to come out (similar to opening a soda bottle). Keep the system at steady pressure, and vent slowly when needed (Bubbles in Microfluidics: How They Form and How to Avoid Them) (Bubbles in Microfluidics: How They Form and How to Avoid Them).
- Design considerations: Microfluidic designers sometimes include bubble traps – side chambers or expansions where bubbles preferentially go and get stuck, protecting the main channel. If your chip doesn’t have one, you can sometimes create an external bubble trap in-line (a piece of wider tubing or a small chamber before the chip). Also, avoid designs with sharp corners or dead-end chambers that are hard to wet – those tend to trap pockets of air (Bubbles in Microfluidics: How They Form and How to Avoid Them).
- Surfacing and wetting: If the channels are hydrophobic and you’re flowing aqueous solutions, pre-treat the channels to make them wettable (plasma treat or flush with ethanol then water (What would be the best way to wet microfluidic device in order to get …)). A completely unwetted hydrophobic channel will allow air pockets to stick. Flushing with 70% ethanol followed by water is a common trick to wet hydrophobic surfaces (ethanol reduces surface tension and helps replace air in the channel with liquid) (What would be the best way to wet microfluidic device in order to get …).
- Bubble removal: Despite all prevention, if a bubble appears, you have a few options. If possible, stop flow and re-prime the chip with high flow to try to push the bubble out to an outlet. Tapping the chip or applying a quick pressure pulse can sometimes dislodge a stubborn bubble (Avoid air bubbles during your microfluidic experiments – Elveflow). Some systems allow you to reverse flow briefly to pull bubbles out. In worst cases, you might have to flush the chip completely and start over. Keep in mind that PDMS is gas-permeable, so small bubbles may eventually dissolve back into PDMS or liquids if flow is stopped and the system is left under pressure (but this can take time).
Contamination and Sample Carryover: Because microfluidic channels have a high surface-to-volume ratio, any contaminants (dust, leftover chemicals, previous sample) can have outsized effects. A speck of dust can clog a 50 µm channel. Residue from a prior experiment can leach into the next one.
- Chip cleanliness: Always use clean chips. Rinse new chips with a bit of solvent or water to remove any debris from fabrication. If reusing a chip, follow a cleaning protocol (see next section on cleaning). Even between runs on the same day, flush the chip with a cleaning solution if the previous sample could interfere with the next.
- Filters: It’s often wise to put a filter in-line with your inputs. For example, using a 5 µm or 0.8 µm syringe filter on sample inputs will remove particulate matter that could clog the chip. Some microfluidic systems have filter membranes at inlets for this reason. Be cautious that filters don’t introduce bubbles (prefill them).
- Avoid cross-contamination: If running multiple samples through the same chip (sequentially), you must wash out the chip thoroughly in between. Because microfluidic channels can trap small volumes in corners or dead-legs, flush with a generous volume of cleaning solution. For instance, running a buffer with some detergent can help rinse out adsorbed substances. However, note that some dead volume might never fully flush if the flow path doesn’t go through it (Internal volume, swept volume, dead volume in microfluidics). In such cases, it might be better to use a fresh chip for a new sample to be safe.
- Dead volume and sample loss: Dead volume refers to fluid that remains in the system but not in active flow paths – e.g., in a connector, a big port, or a stub of tubing. This volume of sample is essentially lost (not utilized) and can also contaminate subsequent runs if not cleared (Internal volume, swept volume, dead volume in microfluidics). To minimize sample loss:
- Use small-volume connectors/fittings (as mentioned, low-dead-volume designs).
- After a run, flush out dead volumes or at least account for them – for example, if a port holds 5 µL of fluid, the first 5 µL of a new sample you inject will just push out the old fluid, not actually reach the channels. You might discard that first part of output as waste.
- Design your experiment so that you don’t rely on recovering extremely small leftover volumes. If only 90% of your sample makes it through to collection because 10% stays in the system, plan for that loss.
- If possible, integrate on-chip valves to push or isolate fluids so that you can recover most of the sample or at least know where it goes.
In practice, a good habit is to examine your chip under a microscope during and after flow. You can see if any bubbles are stuck or if any channel has unexpected residues. Brightfield microscopy will show you particles or bubbles as they flow. If you see a problem, pause and address it then rather than continuing and losing an entire experiment’s worth of data.
To illustrate, consider an example: you’re running a microfluidic immunoassay and switching between different samples on the same chip. To avoid carryover, you might flush with a buffer + 1% bleach solution for a few minutes, then with pure buffer. This can effectively clean protein residues. But you also must ensure all that bleach is gone before the next sample (so a further rinse with a quenching agent or more buffer might be needed). Awareness of what’s happening inside those tiny channels is key – and when in doubt, err on the side of over-cleaning and bubble-checking.
Optimizing On-Chip Sample Loading and Imaging
Once your fluidics are connected and bubble-free, you need to actually load samples/reagents and possibly observe what’s happening in the chip (via microscopy or other detectors). Here are some tips to optimize these steps:
Sample Loading and Injection:
- Precise metering: In microfluidics, small volume differences matter. Use calibrated pipettes or syringe pumps to load exact volumes if needed. Some chips have on-chip volume metering structures (like chambers of known volume). If not, you controlling the injection time or pump volume is important to know how much sample entered.
- Avoiding introduction of bubbles during loading: If you are manually pipetting into a chip reservoir or well (some chips have open wells rather than tubing connections), do so slowly and at a low angle to avoid trapping air. Ensure the inlet well is already filled with buffer so that when you add sample it pushes fluid into the channel rather than air.
- Capillary fill vs. forced injection: Some chips can simply be filled by capillary action (especially if they have open ports). This can be gentle on samples (no pressure needed) but might be slow or not reach all areas if not designed for it. Often a slight pressure or vacuum helps draw the sample in fully. For example, you can apply a gentle vacuum on the outlet to suck sample through once it’s been added to the inlet.
- Consistent flow for replicates: If you are doing multiple runs, try to keep conditions the same for each – e.g., same loading pressure, same wait times. Microfluidic results can be sensitive to flow conditions. A stable pump (or even a simple gravity-driven flow using a fixed-height reservoir) can ensure each run sees the same flow profile.
- Sample/reagent ordering: Plan the sequence of loading if multiple inputs are involved. For instance, in a cell sorting chip you might first fill all channels with buffer, then introduce cell sample in one inlet and sheath fluid in another, starting the flows at the correct ratio. Having a written protocol or checklist is very helpful, since juggling tiny volumes and multiple connections can be error-prone.
Imaging and Detection:
- Microscopy alignment: Mount your chip on a microscope stage securely. If using an upright microscope, you might need a transparent window on one side of the chip or a mirror beneath a translucent chip. Inverted microscopes are more common for microfluidics because you can treat the chip like a slide. Ensure the chip is flat; PDMS chips can sometimes sag or deform – clamping it between glass slides can help keep it planar for imaging.
- Optical clarity: As noted, materials like PDMS and glass are great for imaging. If your chip is plastic, check for any autofluorescence under your imaging conditions beforehand (you can run a blank and see background). If autofluorescence is an issue, you might need to adjust filter sets or use an optical background subtraction. Choosing a low-autofluorescence material like COC can vastly improve fluorescence imaging signal-to-noise (Considerations When Switching from PDMS to Thermoplastic Microfluidics).
- Microscope objectives: Use appropriate objectives for the chip thickness. For example, if you have a 1 mm thick glass slide under your channels, a standard 40x objective will work. But if you have a thick plastic device, you might need a long working distance objective to focus into the channel. For high-NA imaging (oil immersion), it’s best if the channel is right under a thin coverglass. Some people will bond a coverglass onto a PDMS chip specifically to allow high-NA microscopy. Make sure your objective doesn’t crash into the chip – it’s easy to forget the chip is thicker and accidentally bump it while focusing.
- Illumination and detection integration: If you’re using something like a laser or LED to detect signals in the chip (say laser-induced fluorescence or optical sensors), align those carefully and shield against stray light. Many microfluidic chips incorporate optical detection points where the channel might widen or have a reflective surface. Use those features if available. Also consider the refractive index mismatch – e.g., light might refract at a PDMS-air interface, so fill any void with a matching medium or use an immersion objective.
- On-chip sensors: In some setups, you might integrate electrodes or thermometers. Ensure wiring and connections for those don’t impede your optical access or fluid connections. Plan the geometry so you can simultaneously flow and measure what you need.
Workflow Optimization:
- If you are doing a lot of runs (e.g., high-throughput screening on a chip), consider automating parts of the process. Autosampler syringes or valves could load sequences of samples. Robotic stages can move the chip or objectives for multiplexing fields of view.
- Keep the chip accessible. If something goes wrong (like a bubble sneaks in), you want to be able to intervene. It’s frustrating if your chip is buried under a maze of tubing and clamps such that you can’t quickly fix an issue.
- Temperature control: If your experiment needs a certain temperature (cell culture at 37°C or a PCR chip cycling), integrate that smoothly. You might have the chip on a thermal plate or use an incubator that fits your microscope. Remember that temperature changes can also induce bubbles (heating decreases gas solubility – bubbles may form), so degassing is extra important if heating.
One example of loading strategy: In an organs-on-chip device, researchers often prime the chip with media first, then slowly introduce cells at one inlet while drawing fluid from the outlet at a matching rate. After cells are loaded and attached, fresh media is perfused. This multi-step loading (prime → cell seeding → static incubation → flow) ensures no bubbles and that cells distribute correctly.
Another example: In droplet microfluidics, two or more streams (aqueous sample and oil) need to intersect at a junction to form droplets. The flow rate ratio is critical for droplet size. Using high-precision pressure pumps, you can dial in those rates and observe droplets under a microscope in real-time, adjusting as needed. Stable flow (no pulsation) is key to uniform droplet generation (Prototyping Microfluidics vs. Manufacturing Them – uFluidix).
In short, treat your microfluidic experiment as you would a delicate analytical instrument – handle samples carefully, calibrate volumes and flows, and take care to optimize the observation method. With practice, loading and running a microfluidic chip can become as routine as running a gel, but it requires initially paying close attention to details that in the macro world we might ignore (like a tiny bubble or a 1 µL volume difference).
Cleaning and Reusing Microfluidic Chips
Not all microfluidic chips are meant to be reused – many commercial ones are disposable. But for custom or expensive chips (and in research), you often will clean and reuse chips multiple times. Proper maintenance extends the chip’s life and ensures reproducible performance over time.
General Cleaning Approaches:
- Fluid flushing: The simplest cleaning is to flush the channels with a sequence of cleaning solutions. A common protocol: flush with a detergent solution (e.g., 1–2% Alconox or mild lab detergent) to remove organic matter, then flush with water, then perhaps a solvent like isopropanol or acetone to remove organic residues, and finally rinse with deionized water (Efficient cleaning of a microfluidic chip – Chips and Tips). Each step should be flowed for a few minutes to ensure contact with all surfaces. Using an ultrasonic bath with the cleaning fluid circulating through the chip can help dislodge particles (Efficient cleaning of a microfluidic chip – Chips and Tips). For example, you can immerse the chip in a beaker of water in an ultrasonic cleaner while pumping cleaning solution through it – the cavitation assists cleaning (just be careful not to delaminate bonded layers with very strong sonication).
- Chemical cleaning: Depending on what’s stuck in the chip, you may need stronger cleaners. For removing organic residues or polymerized substances, a soak or flush with solvent (acetone, etc.) might work for solvent-resistant chips (glass, some plastics). For biological residues, diluted bleach (sodium hypochlorite) can oxidize organic matter; after bleach, a thorough rinse is needed. Piranha solution (sulfuric acid + hydrogen peroxide) is a very strong cleaner for glass/silicon (it will dissolve organics) but it’s extremely dangerous to use and will destroy PDMS and many plastics – use only in extreme cases and proper environment. A safer alternative is hydrogen peroxide or enzymatic cleaners for protein removal.
- Plasma or UV cleaning: For PDMS chips, sometimes putting the chip in a plasma cleaner (oxygen plasma) for a few minutes can burn off residual organics and also restore surface hydrophilicity (The Practical Guide to Cleaning Microfluidic Devices). UV-Ozone cleaners similarly can clean surfaces at a molecular level. However, note that plasma will also modify surfaces (which might be good or bad for your next experiment) and too long exposure can age PDMS or plastic surfaces.
- Autoclaving: If chips need to be sterile (cell culture applications), many can be autoclaved (121°C steam) if they are made of glass, certain thermoplastics, or PDMS (PDMS autoclaving is generally okay, though it may induce some deformation if not supported, and it will drive out trapped air which can cause bubbles later). Autoclaving also helps clean some contaminants. But autoclaving can craze some plastics (e.g., polycarbonate will cloud). Ethanol sterilization is another route for disinfection.
PDMS-Specific Issues: PDMS devices, when reused, tend to absorb whatever was in them. For example, dyes or drugs might tint the PDMS. Plasma can help remove some, but often PDMS devices are considered semi-disposable for this reason. If you need to reuse PDMS, try to bake it at ~80°C for a few hours to outgas absorbed solvents, and store it dry. Storing PDMS chips in water can actually grow algae or bacteria over time (since PDMS is slightly porous and can let in nutrients); if you store in water, add a bit of biocide or keep refrigerated.
Glass/Plastic Chip Reuse: Rigid chips (glass, hard plastic) can often be cleaned effectively and reused dozens of times if handled carefully. For glass microfluidic devices, a cleaning protocol akin to glassware cleaning works: detergent, water, maybe a dilute acid wash, water, then dry. An efficient method reported is using ultrasonic cleaning in plastic bags: put the chip in a bag with cleaning solution, seal it, then sonicate – this avoids dirtying the sonicator bath and seems to improve cleaning consistency (Efficient cleaning of a microfluidic chip – Chips and Tips) (Efficient cleaning of a microfluidic chip – Chips and Tips).
When to discard: No matter how well you clean, eventually chips may degrade:
- PDMS bonding can weaken and start leaking.
- Plastic chips might develop cracks or scratches.
- Channels could become permanently fouled (e.g., coated with adsorbed polymers or clogged with precipitate you can’t remove).
If you notice performance declining (strange flow patterns, high pressure, cells not sticking like they used to, etc.), it may be time to use a new chip. Keep records of how many times a chip has been reused and under what conditions; you might find, for instance, that a PDMS chip is reliable for ~10 uses before something changes.
Maintenance between runs:
- If you plan to reuse soon, you can keep the chip filled with a storage solution (e.g., fill with 70% ethanol or sterile buffer to prevent microbial growth, and cap the ends).
- Keep the chip dust-free. Store it in a clean petri dish or container. Dust particles can enter ports and later become clogs.
- For devices with integrated electrodes or sensors, follow any specific instructions (e.g., don’t use strong acid if you have metal electrodes that could corrode; don’t autoclave if it could damage sensors).
Example cleaning protocol: A group using a protein analysis chip (glass/PDMS) might do the following after each run: inject 1% Triton X-100 (detergent) and leave for 5 minutes, flush with water, inject 0.1 M HCl and leave 1 minute (to dissolve any calcified deposits), flush with water thoroughly, then flush with isopropanol, then dry with air. Finally, store the chip in a dry, covered container. This sequence removes biological residues and reactivates surfaces to a clean state.
Another example: For a cell culture organ-on-chip, one might perfuse a warm enzymatic cleaner (like trypsin or protease solution) to detach any remaining cells and break down extracellular matrix, then flush with disinfectant. If reusing for cells, a sterilization step (like UV exposure in a biosafety hood for 30 min) could be added.
The key is to validate your cleaning. If possible, run a blank test after cleaning – e.g., push pure buffer through and see if you detect any signal from previous samples. Or microscope-inspect the channels to ensure no debris remains. Good maintenance ensures that one chip can serve through many experiments without causing mystery artifacts.
Conclusion
Integrating microfluidic chips into the lab may initially seem daunting, with all the tubing, connectors, and potential pitfalls like bubbles. However, with careful setup and practices, a microfluidic experiment can run as smoothly as any benchtop assay, yielding reliable and high-quality data. By selecting appropriate interfacing hardware, rigorously preventing contamination and bubbles, optimizing loading/imaging, and maintaining your devices, you can fully harness the power of microfluidics in your research workflow.
Every lab setup is unique – you might have specific pumps, microscopes, or analysis needs. Custom-designed microfluidic chips can be created to seamlessly integrate with your existing equipment. Imagine a chip that has the same port connectors as your HPLC, or a device sized to fit exactly on your microscope stage, or one with built-in bubble traps tailored to your application. Our team specializes in designing such bespoke microfluidic solutions. If you want your microfluidic system to plug-and-play into your lab, reach out to us. We can collaborate to design and fabricate chips with the right interfacing, geometry, and features to match your workflow, ensuring that you spend less time troubleshooting and more time collecting data. Let us help you take the guesswork out of microfluidic integration – contact us to discuss your needs and get started on a custom microfluidic chip that will streamline your research.
See more of Our Posts
Microfluidics category
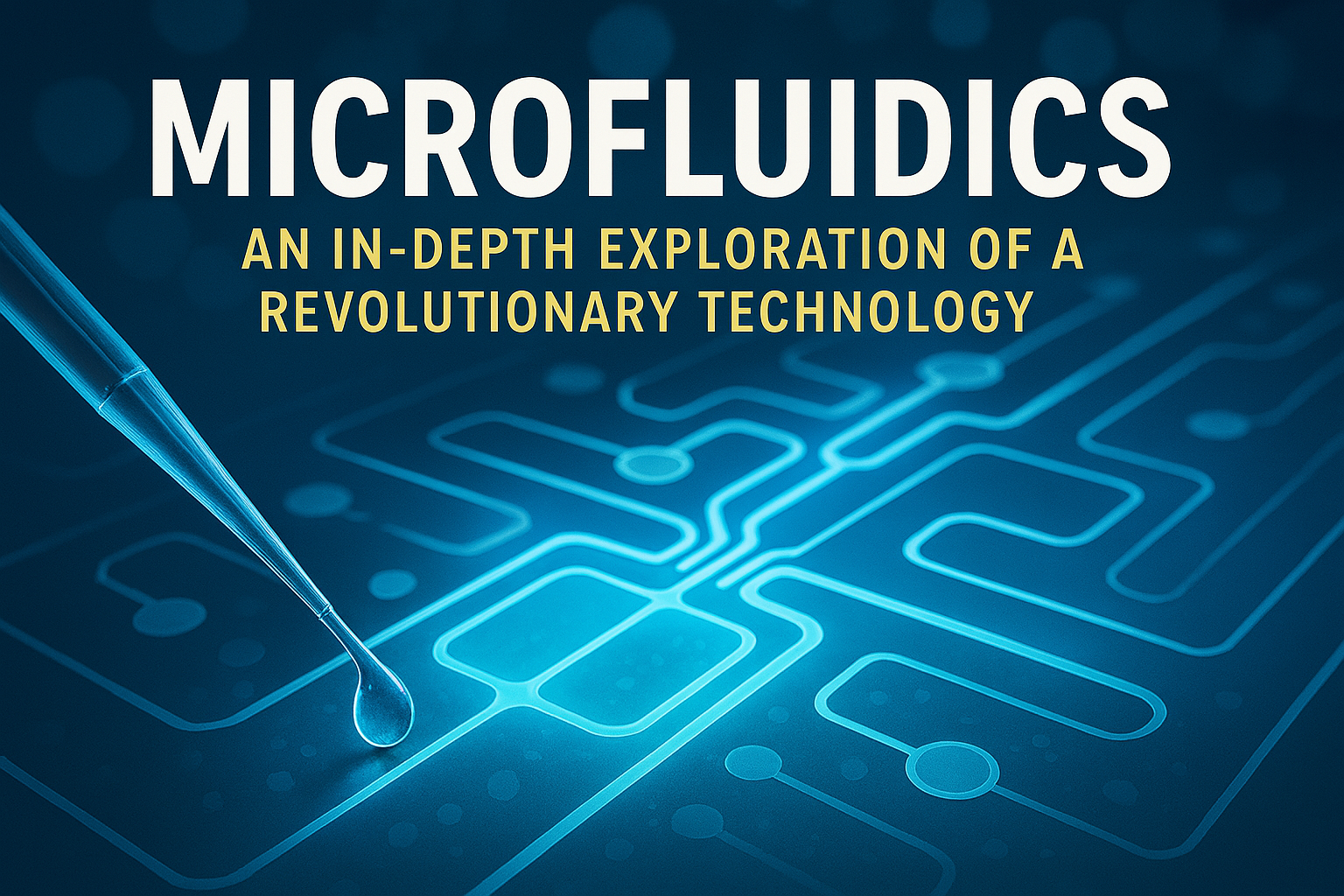
Microfluidics in Research: A Complete Reference on Design, Fabrication, and Applications
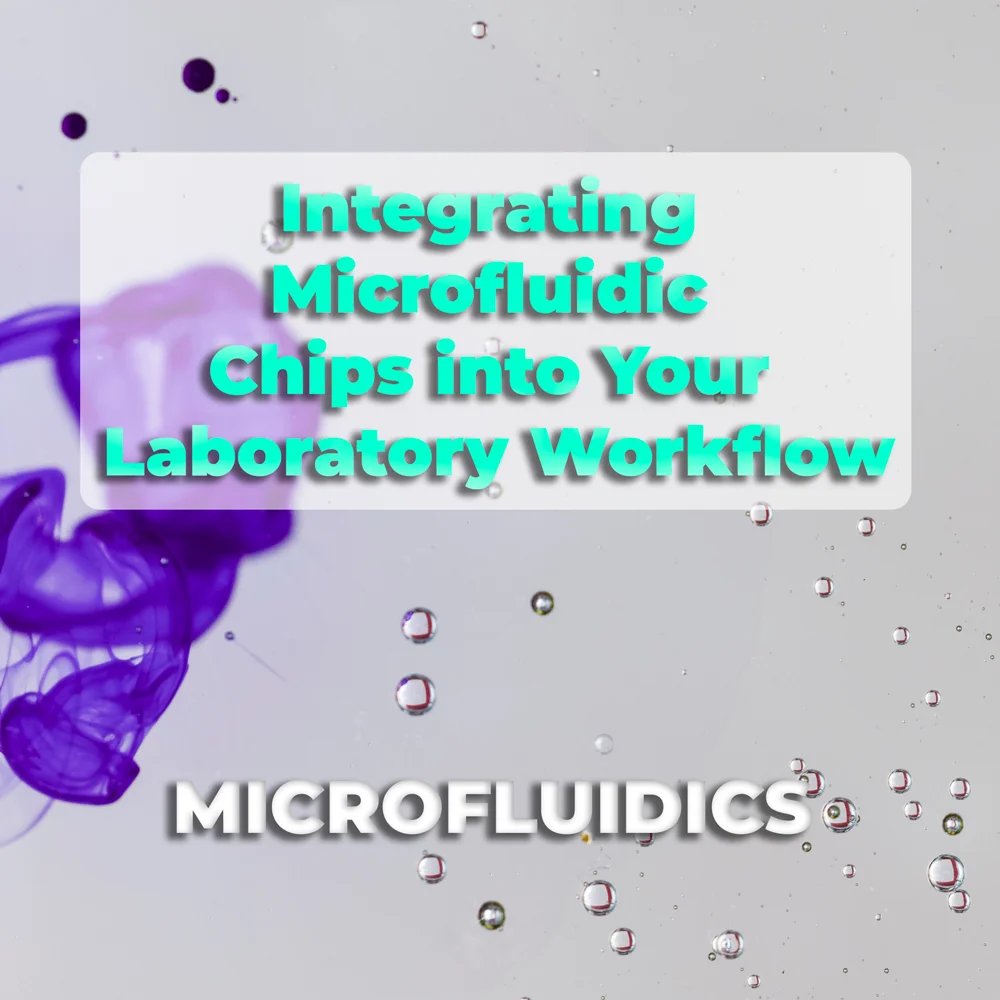
Integrating Microfluidic Chips into Your Laboratory Workflow
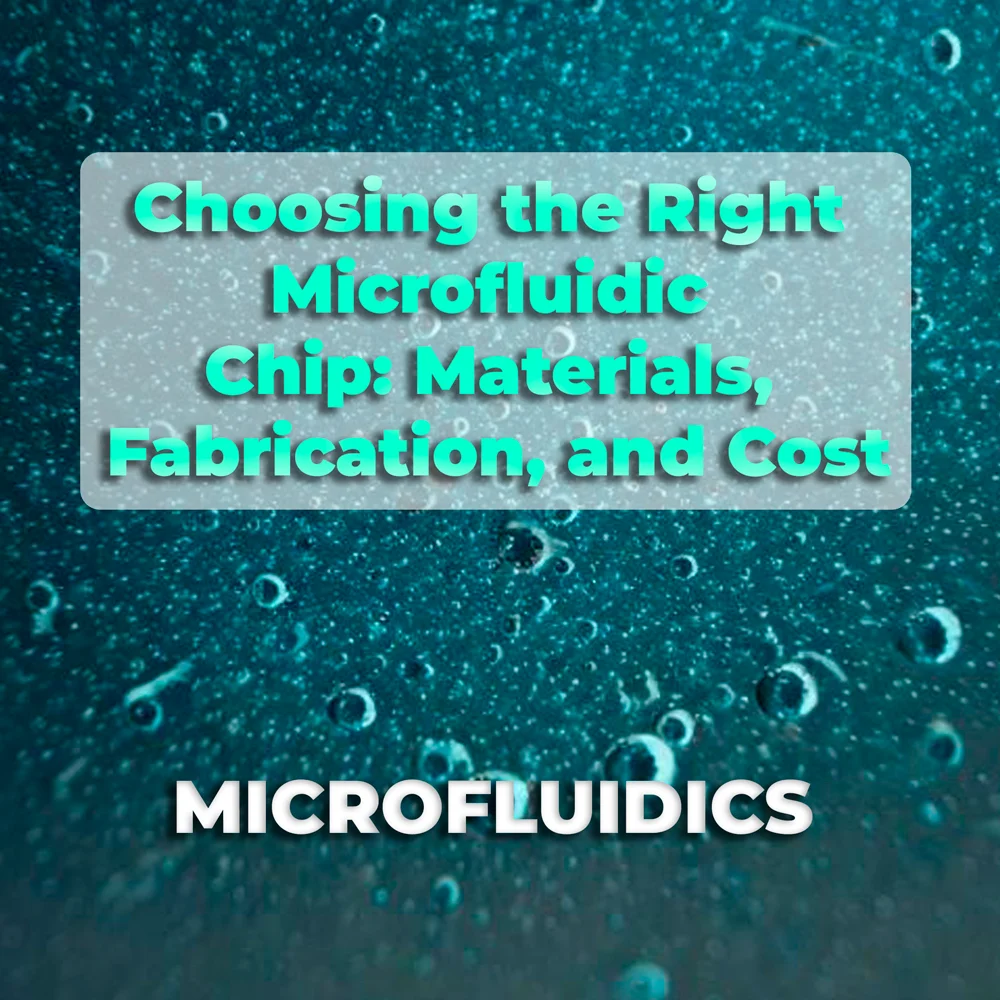
Choosing the Right Microfluidic Chip: Materials, Fabrication, and Cost
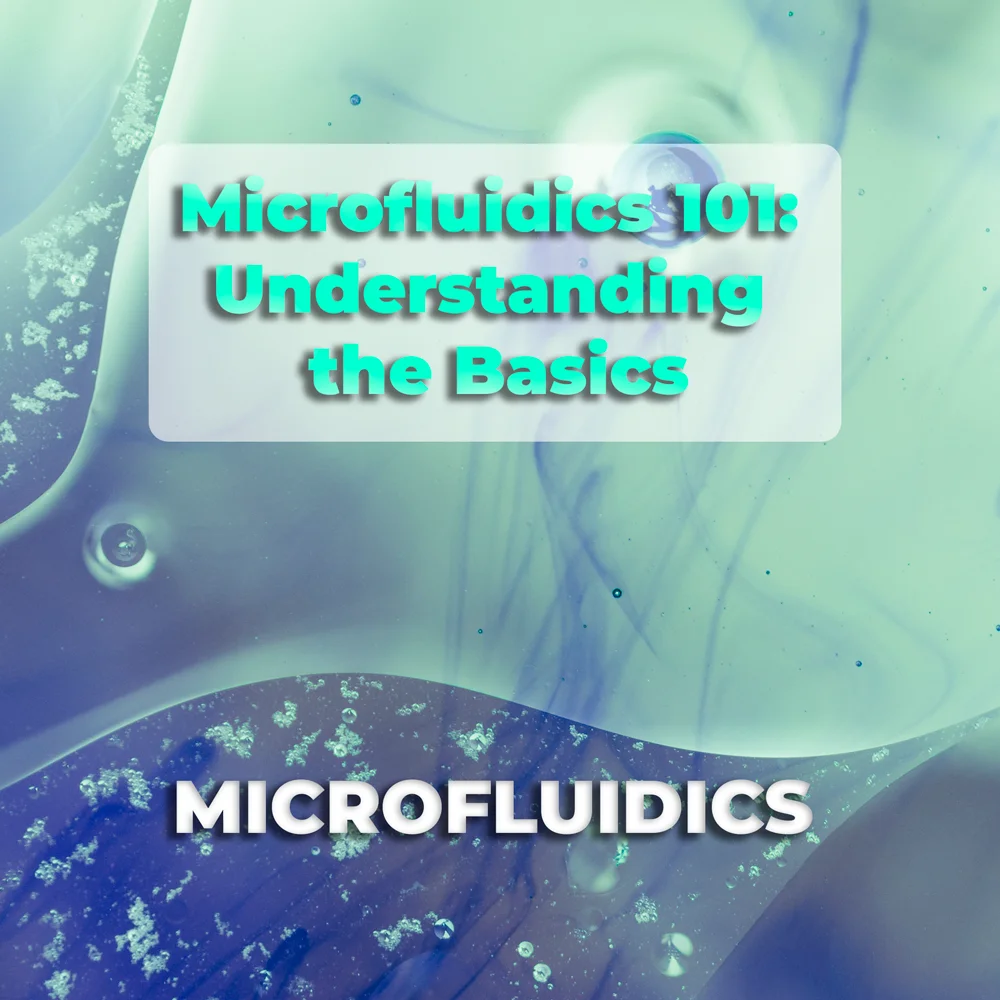
Microfluidics 101: Understanding the Basics
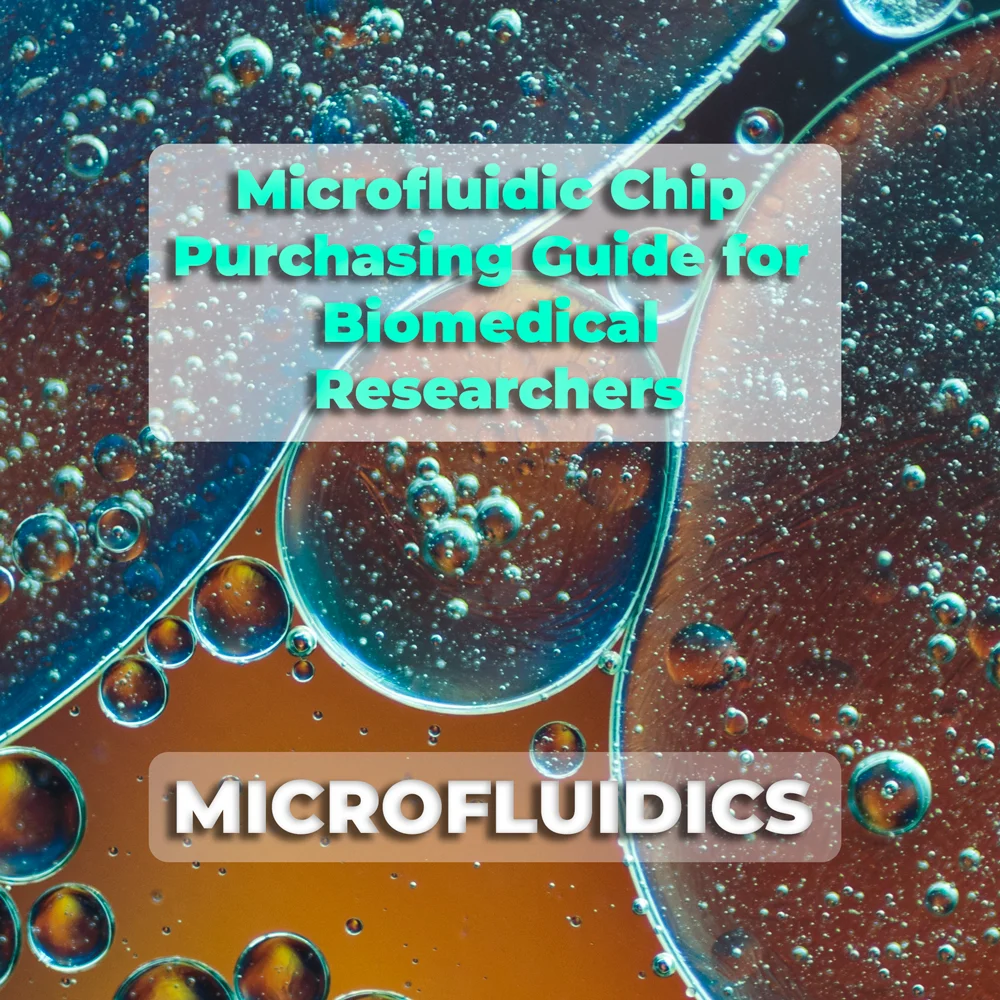
Microfluidic Chip Purchasing Guide for Biomedical Researchers
All Resources
Never miss our posts!
Author:
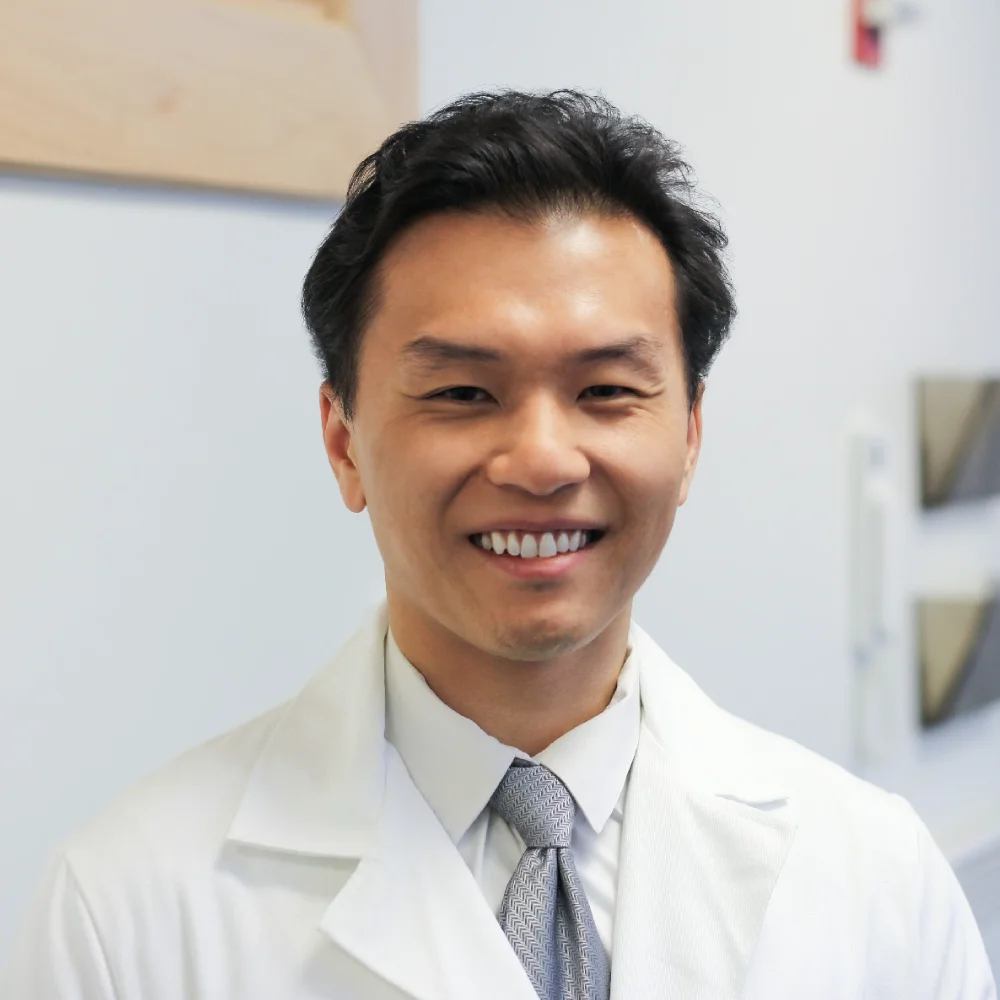
Shuhan He, MD
Shuhan He, MD is a dual-board certified physician with expertise in Emergency Medicine and Clinical Informatics. Dr. He works at the Laboratory of Computer Science, clinically in the Department of Emergency Medicine and Instructor of Medicine at Harvard Medical School. He serves as the Program Director of Healthcare Data Analytics at MGHIHP. Dr. He has interests at the intersection of acute care and computer science, utilizing algorithmic approaches to systems with a focus on large actionable data and Bayesian interpretation. Committed to making a positive impact in the field of healthcare through the use of cutting-edge technology and data analytics.