Introduction
Ultracentrifugation is a specialized technique used to spin samples at exceptionally high speeds. Current ultracentrifuges can spin to as much as 150 000 rotations per minute (rpm) (equivalent to 1 000 000 g) (Biocompare, 2019b). However, extreme centrifugal forces may cause overheating, so to avoid sample damage, ultracentrifuges are equipped with vacuum systems that keep a constant temperature in the centrifuge’s rotor (Biocompare, 2019b).
Centrifugation, and ultracentrifugation, is nowadays, at the core of the laboratory routine. Benchtop centrifuges are essential devices in any biology or chemistry laboratory, and they are used on a day-to-day basis in a wide range of experimental protocols, from concentrating solutions to isolating cells and subcellular components. Ultracentrifugation widened the applications of benchtop centrifugation, allowing the isolation of smaller sized particles, and the study of purified molecules and molecular complexes (Ohlendieck & Harding, 2017). In biology, the development of ultracentrifugation in the early 1900s, widened the possibilities of scientific research to the subcellular level, allowing for the differential separation of cellular components, such as organelles, lipid membranes, and even to purify proteins and ribonucleic acids (DNA and RNA).
The Principle of Ultracentrifugation
The basis of ultracentrifugation is the same as normal centrifugation: to separate the components of a solution based on their size and density, and the density (viscosity) of the medium (solvent) (Ohlendieck & Harding, 2017). As a general principle, (ultra)centrifugation abides by the following rules (Ohlendieck & Harding, 2017):
- the denser a biological structure is, the faster it sediments in a centrifugal field
- the more massive a biological particle is, the faster it moves in a centrifugal field
- the denser the biological buffer system is, the slower the particle moves in a centrifugal field
- the greater the frictional coefficient (i.e., the friction between the component and the neighboring environment) is, the slower a particle moves
- the greater the centrifugal force is, the faster the particle sediments
- the sedimentation rate of a given particle will be zero when the density of the particle and the surrounding medium are equal.
Centrifugation Versus Ultracentrifugation
The use of ultracentrifugation over centrifugation (and vice-versa) denotes basic differences between the two techniques. Fundamental differences between centrifugation and ultracentrifugation include:
- Spinning velocity, and therefore, the centrifugal force applied to the samples. The rotor of an ultracentrifuge can spin as high as 1 000 000 x g, while most common benchtop centrifuges are limited to 65 000 x g (Biocompare, 2019a, 2019b). This brings up the second basic difference:
- Refrigeration and vacuum systems, are mandatory in ultracentrifuges. Because of the extremely high spinning speed, ultracentrifuges are always equipped with vacuum and refrigeration systems, to avoid sample and/or device damage due to frictional force and overheating. In benchtop centrifuges, these two systems are optional, with the most simple centrifuges, like mini microcentrifuges, displaying none.
- Type of pellet that is produced from sample fractionation: because ultracentrifuges can achieve much higher spinning speeds, the type of sediment (pellet) that results from one or the other is also different, with ultracentrifugation allowing for the isolation of smaller particles than benchtop centrifugation. In biology labs, subcellular fractionation to separate cytosolic contents (such as whole cytosol, mitochondria, or chloroplasts) from cell nuclei can be attained by benchtop centrifuges. However, to isolate smaller components such as ribosomes and small vesicles, higher centrifugal forces, only possible with ultracentrifuges, are necessary (Momen-Heravi, 2017; Ohlendieck & Harding, 2017).
Types of Ultracentrifugation: Analytical Versus Preparative
There is, currently on the market, a wide variety of ultracentrifuges. The choice among different brands and models must consider the type of experimental applications to be performed, the availability of different rotors (making it possible to adapt the ultracentrifuge to different experimental settings), and the temperature range. Some ultracentrifuges further offer remote monitoring and control, and password-protection (Biocompare, 2019b).
It is important to ask: For which experimental setting do I need an ultracentrifuge? In that sense, two types of ultracentrifuges are available: analytical and preparative. Analytical ultracentrifugation is used in the study of purified macromolecules or supramolecular assemblies, while preparative ultracentrifugation is used in the actual separation of tissues, cells, subcellular components, and other biochemically interesting particles (Ohlendieck & Harding, 2017).
Analytical ultracentrifuges are equipped with optical detection systems that allow the researcher to follow the centrifugation process in real-time. These systems may use ultraviolet (UV) light absorption or refracting index interference (RII) optical detection systems (ultracentrifuges may be equipped with one or both types of optical systems) (Cole, 2009). While UV detection directly measures the absorbance (abs) of a substance at a specific wavelength, RII measures changes in the refraction index (radiation direction) of a given substance, compared to the solvent it is dissolved in (Ohlendieck & Harding, 2017). The purpose of analytical centrifugation is different from other types of centrifugation. Although component isolation is possible with analytical centrifugation, the goal of this technique is to obtain data to characterize the sample that is spun (sedimentation velocity, viscosity, concentration, etc.). With analytical centrifugation, it is possible to follow the variations in sample concentration as a function of the applied centrifugal force. This technique is used in two main experimental settings: sedimentation velocity and sedimentation equilibrium studies, which are key in macromolecular characterization (Ohlendieck & Harding, 2017). Results from sedimentation velocity experiments provide data that are used to calculate the molecular size (molecular weight), shape, and molar masses of new chemical molecules, ribonucleic acids, proteins, and others (Cole, 2009; Ohlendieck & Harding, 2017).
Preparative ultracentrifuges are mostly used to process biological samples for further analysis. The most common application of preparative ultracentrifugation is in tissue and subcellular fractionation, to isolate increasingly smaller components of the biological samples (Jasinski, Schwartz, Haque, & Guo, 2015; Martin et al., 2018; Momen-Heravi, 2017; Ohlendieck & Harding, 2017; Wasan, Cassidy, Kennedy, & Peteherych, 2001). For that, two main centrifugation methods are used: differential and density-gradient centrifugation.
- Differential centrifugation is used to separate the components of a solution based on differences in the sedimentation rate of the different components of the mixture. As explained above (see section 2: The Principle of Ultracentrifugation), the sedimentation properties of a substance depend on its size and density but also on the density of the solvent. In medical and biology labs, crude tissue homogenates containing organelles, membrane vesicles, and other structural fragments are divided into different fractions by the stepwise increase of the applied centrifugal field. Furthermore, differential centrifugation is also routinely used in the isolation of non-living substances, like nanoparticles, colloids, and viruses (Long, Borsa, & Sargent, 1976). The general principle of differential centrifugation is outlined in Figure 1.
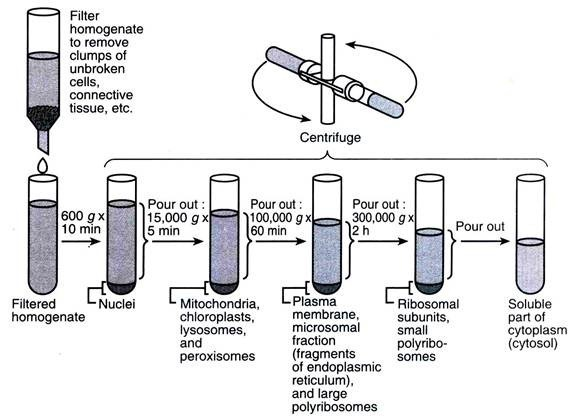
Figure 1: General principle of differential centrifugation, applied to subcellular fractionation (image credit: (Kumar))
Density gradient centrifugation goes further in particle separation than differential centrifugation. It is ideal when the goal is to isolate particles of similar sizes, but different densities. In this case, it is possible to establish density gradient solutions with increasing concentrations of specific materials, in the spinning tubes (Figure 2). Cesium salt gradients are used in the separation of DNA, and sucrose gradients are used in subcellular fractionation to isolate organelles and multiprotein complexes, like ribosomes (Dumetre & Darde, 2004; Perper, Zee, & Mickelson, 1968). Today, there are several commercial gradient solution kits to isolate specific particles. Roughly, there are two types of density gradient centrifugation: rote-zonal centrifugation and isopycnic centrifugation (also called equilibrium centrifugation), which differ in the way particles are separated across the gradient (see Figure 2).
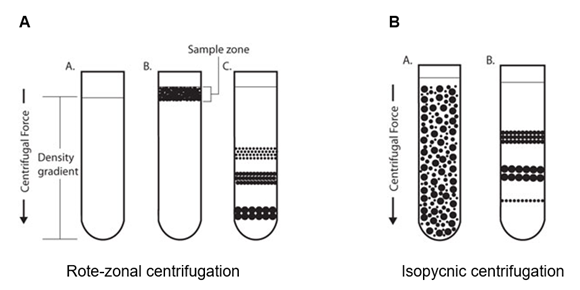
Figure 2: Types of preparative centrifugation. A – Rote-zonal centrifugation B – Isopycnic centrifugation (image credit: (Frei))
Rote-zonal centrifugation – particle separation depends mostly on particle mass. Zones, or bands, are generated, each containing a particle fraction of a specific mass. However, care must be taken when performing rote-zonal centrifugation. Because the mass of the particles is higher than the density of the solvent, if they are centrifuged for too long, all particles will eventually deposit in the bottom of the tube (Frei).
Isopycnic (equilibrium) centrifugation – particle separation depends solely on their density. In isopycnic separation, particles are mixed with the gradient solution, and during centrifugation, they will move until they reach the gradient phase which equals their density (isopycnic or equilibrium point). Because the density of the gradient medium is always higher than the density of particles, these will never sediment, independently of the centrifugation time. Continuous gradients may be used in isopycnic centrifugation, however, discontinuous gradients in which particles form bands at the interface between the density gradient layers are more suitable for the separation of some biological samples, like the separation of lymphocytes from whole blood (Frei; Ohlendieck & Harding, 2017).
Applications of Analytical and Preparative Ultracentrifugation
Due to their intrinsic differences, analytical and preparative ultracentrifugation are used for different purposes:
Analytical ultracentrifugation
- determination of the purity (including the presence of aggregates) and oligomeric state of macromolecules, by recording sedimentation velocity data
- determination of the average molecular mass of solutes in their native state
- Study of changes in the molecular mass of supramolecular complexes,
- using either sedimentation velocity, sedimentation equilibrium (or both)
- the detection of conformation and conformational changes
Preparative ultracentrifugation
- subcellular fractionation
- affinity purification of membrane vesicles
- separation of DNA components
- colloid separation
- virus purification
The Ultracentrifuge: How to Use and How to Care
Modern ultracentrifuges are heavy, sturdy equipment that requires certain know-how for proper usage and care.
- Rotor balance. As in all centrifuges, sample spinning requires a proper balance of the weight inside the rotor. Given the extremely high spinning speed inside the ultracentrifuge’s rotor, the impact of subtle imbalances may be shockingly strong. Modern ultracentrifuges have some buffer capacity, to absorb slight weight imbalances, and when there is too much imbalance, an automatic system shuts off the device. Moreover, in all ultracentrifuges, the rotor is encapsulated in a strong heavy metallic cage, to avoid vibrations and projections that could damage the sample and endanger the operator. Yet, it is of vital importance that the ultracentrifuge is properly loaded, according to the manufacturer’s instructions.
- Sample position in the rotor. All rotor positions must be filled. Even when there are only a few tubes, the rest of the positions must be occupied with blank samples of equivalent weight. To avoid both rotor and sample damage, it is important to set the ultracentrifuge to slow acceleration and deceleration modes. This is especially important in density gradients, as the sudden stop of the spinning may affect the separation of the gradient layers (Ohlendieck & Harding, 2017). Ultracentrifuges are expensive devices, which are required to accurately separate particles in solution. To ensure the proper function of the ultracentrifuge, care measures must be undertaken regularly. Apart from safety, proper loading of the rotor avoids excessive vibration, which can cause damage to the device.
- Centrifuge cleaning. Maintenance and cleaning of the rotor must be done with non-abrasive detergents to avoid corrosion. Rotor cleaning is especially important to ensure that there are no remnants of the samples that were centrifuged, and therefore, should always be performed after spinning.
- Storage. Whenever the device is not used, or simply for overnight storage, rotors must be kept in a dry room, properly cleaned, and left to dry in an inverted position, to avoid the accumulation of water in the sample cells.
- Regular maintenance. This should be done by certified operators to ensure the proper long-term function of the ultracentrifuge.
Advantages and Limitations of Ultracentrifugation
From the development of the first ultracentrifuge in the 1920s by Svedberg, up to today, the scientific advances that resulted from the application of ultracentrifugation to biology, chemistry, material science, and others, are countless. In its most obvious approaches, ultracentrifugation extended the limits of biology research to the subcellular level, by allowing the isolation of particles as small as ribosomes, subcellular organelles, membranes, and ribonucleic acids. With the advent of analytical ultracentrifugation, research took another step further towards the understanding of the submicroscopic world, with the ability to further characterize molecular size, shape, and structure. However, ultracentrifugation has its own limitations, like any other laboratory technique. These include:
- Low sample yield – In preparative ultracentrifugation, samples must be washed several times after spinning, to ensure that there is no cross-contamination between fractions. Samples for preparative centrifugation are usually limited in size (e.g., tissues) or volume (e.g., cell suspensions or blood). In every wash step that a sample is subjected to, there is a loss of material, and thus, after an ultracentrifugation protocol, the yield can be very low.
- Ultracentrifugation is still a time-consuming process, and it can take up to several hours to fractionate all the components of a single mixture.
- Ultracentrifuges are extremely expensive devices, which require constant maintenance, therefore ultracentrifuges are not routinely found in labs, but there is usually only one device per department or university.
Conclusion
To date, ultracentrifugation, in its most varied forms and protocols is used routinely and continues to be a fundamental approach not only in academic research but also in industry and the medical context. Nevertheless, it is fundamental to keep in mind how to properly use ultracentrifuges, to keep the sample and device user safe, and to ensure long-term functionality of the devices.
References
- (2019a). Laboratory Centrifuges. Retrieved November, 2019, from https://www.biocompare.com/Lab-Equipment/Laboratory-Centrifuges/
- (2019b). Ultracentrifuges. from https://www.biocompare.com/Lab-Equipment/10155-Benchtop-Ultracentrifuge/
- Cole, J. L. (2009). Analytical ultracentrifugation. In I. D. Wilson, E. R. Adlard, M. Cooke & C. F. Pole (Eds.), Handbook of methods and instrumentation in separation science (Vol. 1, pp. 34-41). Academic Press.
- Dumetre, A., & Darde, M. L. (2004). Purification of Toxoplasma gondii oocysts by cesium chloride gradient. J Microbiol Methods, 56(3), 427-430. doi: 10.1016/j.mimet.2003.11.020
- Frei, M. Centrifugation Separations. Retrieved December, 2019, from https://sigmaaldrich.com/technical-documents/articles/biofiles/centrifugation-separations.html#ref
- Jasinski, D. L., Schwartz, C. T., Haque, F., & Guo, P. (2015). Large scale purification of RNA nanoparticles by preparative ultracentrifugation. Methods Mol Biol, 1297, 67-82. doi: 10.1007/978-1-4939-2562-9_5
- Kumar, P. Methods used for Separation of Particles in Centrifugation: 3 Methods. Retrieved December, 2019, from http://www.biologydiscussion.com/biochemistry/centrifugation/methods-used-for-separation-of-particles-in-centrifugation-3-methods/12453
- Long, D. G., Borsa, J., & Sargent, M. D. (1976). A potential artifact generated by pelleting viral particles during preparative ultracentrifugation. Biochim Biophys Acta, 451(2), 639-642. doi: 10.1016/0304-4165(76)90162-8
- Martin, S. S., Giugliano, R. P., Murphy, S. A., Wasserman, S. M., Stein, E. A., Ceska, R., . . . Sabatine, M. S. (2018). Comparison of Low-Density Lipoprotein Cholesterol Assessment by Martin/Hopkins Estimation, Friedewald Estimation, and Preparative Ultracentrifugation: Insights From the FOURIER Trial. JAMA Cardiol, 3(8), 749-753. doi: 10.1001/jamacardio.2018.1533
- Momen-Heravi, F. (2017). Isolation of Extracellular Vesicles by Ultracentrifugation. Methods Mol Biol, 1660, 25-32. doi: 10.1007/978-1-4939-7253-1_3
- Ohlendieck, K., & Harding, S. E. (2017). Centrifugation and Ultracentrifugation.
- Perper, R. J., Zee, T. W., & Mickelson, M. M. (1968). Purification of lymphocytes and platelets by gradient centrifugation. J Lab Clin Med, 72(5), 842-848.
- Wasan, K. M., Cassidy, S. M., Kennedy, A. L., & Peteherych, K. D. (2001). Lipoprotein isolation and analysis from serum by preparative ultracentrifugation. Methods Mol Med, 52, 27-35. doi: 10.1385/1-59259-073-X:27