Introduction
Karl Lashley, a prominent neuropsychologist of the early 20th century, embarked on a quest to identify a specific brain region responsible for storing learned information, known as the “engram.” His approach involved creating various mazes for rodents, reasoning that if a rat could be trained to navigate a maze, he could then lesion different parts of the brain to pinpoint where that memory was stored [1]. Although Lashley did not succeed in localizing the engram—since we now understand that memories are distributed across cortical regions—his innovative mazes, including the Lashley III, remain valuable tools in the study of learning and memory.
The Lashley III maze, in particular, is widely utilized for low-stress assessments of spatial learning and memory in rodents. This apparatus consists of a starting box, four interconnected alleyways, and a finishing box that can be modified to resemble the animal’s home cage. Rodents are motivated to find the finishing box through the presence of a food reward or by using bedding from their home cage to simulate familiar surroundings. Both stimuli have proven equally effective in motivating subjects to navigate the maze [2][3].
Figure: Lashley III Maze
Throughout several trials, animals gradually improve their ability to navigate the maze more efficiently. Key metrics for assessing this learning process include the time taken to reach the goal and the number of errors, such as wrong turns, made along the way. Additionally, the total distance traveled can be recorded to evaluate the animal’s maze-solving efficiency. This measure may offer a more accurate reflection of efficiency compared to the total time spent in the maze, as travel speed can vary among animals. In animals that are naive or untreated (i.e., those without learning impairments), all these metrics typically decrease with repeated trials.
How to Use the Lashley III Maze Test
When using a food reward in the goal box, it is essential to acclimate the animals to the food pellets before testing begins. One day prior to the first trial, provide the animals with sample food pellets in their home cage to familiarize them with the reward. Following this, place the animals in each of the four alleyways of the testing apparatus for four minutes with the openings blocked. Afterward, place the animals in the goal box along with additional reward pellets for six minutes. This acclimation process helps the animals become familiar with the testing environment and the reward without learning to navigate the maze, thereby reducing novelty-induced stress on the first day of testing [4].
When using a simulated home-cage box as the goal, improved behavioral performance can be achieved by habituating the animals to the simulated home-cage box for two weeks before starting the experimental protocol [5]. Additionally, the same apparatus habituation protocol described above should be followed the day before testing to minimize stress associated with environmental novelty.
Figure: Lashley III maze, showing the start and the goal compartments and the four alleys.
Here’s a step by step guide:
- Maze Setup: The Lashley III maze typically consists of a series of alleys arranged in a specific pattern. The setup should be placed in a controlled environment to minimize external influences.
- Habituation: Before the actual test, subjects are often given a habituation period to get accustomed to the maze environment without any time pressure.
- Training Phase: The rodents are placed at the start point, and the goal is to find the endpoint, which is often associated with a reward such as food. Researchers record the time taken and the number of errors made (wrong turns).
- Testing Phase: After several training sessions, the actual test phase begins. The rodents’ performance is measured and compared across different conditions or groups.
- Data Analysis: Key metrics include the time taken to complete the maze, the number of errors, and the path taken. Advanced tracking software can provide detailed analyses of movement patterns.
What is it Used For?
The Lashley III maze test is primarily used to evaluate cognitive functions such as:
- Learning and Memory: By observing how quickly and efficiently an animal learns to navigate the maze, researchers can infer the state of its memory and learning capabilities [6].
- Spatial Navigation: The test provides insights into how rodents use environmental cues to find their way, shedding light on spatial memory and navigation skills [7].
- Effects of Neuroactive Substances: Researchers often use the maze to study the impacts of drugs, brain lesions, or genetic modifications on cognitive abilities [1].
- Aging and Neurodegeneration: It is a valuable tool in assessing age-related cognitive decline and the effects of neurodegenerative diseases like Alzheimer’s [7].
Disease and Disorder States
Researchers have utilized the Lashley III maze to model learning deficits associated with Alzheimer’s disease and Fragile X syndrome by employing a transgenic mouse model that lacks the Kv4.2 potassium channel (Kv4.2 KO mice). These mice were observed to make more errors while navigating the maze, indicating a deficit in learning acquisition [8].
The Lashley III maze has also been employed to study learning differences between animals of varying social statuses. Chronic stress induced by social domination is linked to poor memory performance. For example, socially subordinate mice, which experience chronic stress from being dominated, exhibit deficiencies in navigating the Lashley III maze. These mice are slower to learn the maze, make more errors, and take longer to reach the goal box compared to their non-subordinate counterparts [9]. Thus, the Lashley III maze is useful for assessing memory deficits associated with stress in addition to those induced by disease states.
Furthermore, the Lashley III maze has been used to evaluate the effects of drugs on memory functions. Cognitive enhancers like nicotine have been shown to improve performance in the maze, as evidenced by accelerated learning in young mice administered the drug [10]. Conversely, the offspring of mice treated with the psychomotor stimulant amphetamine during pregnancy, which is known to impair cognition, show slower rates of maze learning [11].
Neuroanatomy
Performance in the Lashley III maze is heavily influenced by the function of the hippocampus. Comparative studies across multiple mouse strains have revealed that cortical ectopias caused by autoimmune disorders do not significantly affect maze performance in strains without abnormal cortical development, such as the commonly used RF mouse strain.
In contrast, the BXSB mouse strain, an autoimmune disease model that exhibits cortical ectopias, shows no significant performance deficits. However, NZB mice, which have similar cortical ectopias and additional developmental malformations of the hippocampus, perform significantly worse in the maze. These findings suggest that hippocampal function is more critical for maze performance than cortical function, challenging Karl Lashley’s initial hypothesis that cortical function was the primary mediator of learning and memory in this spatial task [1][12].
The Lashley III maze is typically constructed with opaque plexiglass walls to eliminate external visual cues, thereby focusing learning on egocentric motivators like locating food or a home cage. However, the maze can also be configured with transparent walls to incorporate allocentric cues. Under these conditions, researchers have observed increased reliance on the anteromedial extrastriate complex, a brain region associated with spatial learning using external visual cues. This flexibility allows the Lashley III maze to be adapted for studying different types of memory that involve various neuroanatomical regions [13].
By modifying the Lashley III maze to include either egocentric or allocentric cues, researchers can gain insights into the distinct neural circuits and brain regions involved in different types of spatial learning and memory. This adaptability makes the maze a valuable tool for exploring the complexities of neuroanatomy and cognitive function.
References
- Lashley, K. S. (1933). INTEGRATIVE FUNCTIONS OF THE CEREBRAL CORTEX. Physiological Reviews, 13(1), 1–42.doi:10.1152/physrev.1933.13.1.1
- Morris, R. (1984). Developments of a water-maze procedure for studying spatial learning in the rat. Journal of Neuroscience Methods, 11(1), 47-60.
- D’Hooge, R., & De Deyn, P. P. (2001). Applications of the Morris water maze in the study of learning and memory. Brain Research Reviews, 36(1), 60-90.
- Matzel, L. D., Han, Y. R., Grossman, H., Karnik, M. S., Patel, D., Scott, N., … Gandhi, C. C. (2003). Individual Differences in the Expression of a “General” Learning Ability in Mice. Journal of Neuroscience, 23(16), 6423–6433.
- Blizard, D. A., Weinheimer, V. K., Klein, L. C., Petrill, S. A., Cohen, R., & McClearn, G. E. (2006). “Return to Home Cage” as a Reward for Maze Learning in Young and Old Genetically Heterogeneous Mice. Comparative Medicine, 56(3), 196-201.
- Vorhees, C. V., & Williams, M. T. (2006). Morris water maze: procedures for assessing spatial and related forms of learning and memory. Nature Protocols, 1(2), 848-858.
- Barnes, C. A. (1979). Memory deficits associated with senescence: A neurophysiological and behavioral study in the rat. Journal of Comparative and Physiological Psychology, 93(1), 74-104.
- Smith, G. D., Gao, N., & Lugo, J. N. (2017). Kv4.2 knockout mice display learning and memory deficits in the Lashley maze. F1000Research, 5, 2456.
- Colas-Zelin, D., Light, K. R., Kolata, S., Wass, C., Denman-Brice, A., Rios, C., Szalk, K., … Matzel, L. D. (2012). The imposition of, but not the propensity for, social subordination impairs exploratory behaviors and general cognitive abilities. Behavioural brain research, 232(1), 294-305.
- Fisher, A., Hanin, I., Poewe, W., & Windisch, M. (2007). Alzheimer’s and Parkinson’s Diseases. Karger Medical and Scientific Publishers.
- Nasello, A. G., & Ramirez, O. A. (1978). Open-field and Lashley III maze behaviour of the offspring of amphetamine-treated rats. Psychopharmacology, 58(2), 171–173.
- Schrott, L. M., Denenberg, V. H., Sherman, G. F., Rosen, G. D., & Galaburda, A. M. (1992). Lashley maze learning deficits in NZB mice. Physiology & Behavior, 52(6), 1085–1089
- Bressler, A., Blizard, D., & Andrews, A. (2010). Low-stress route learning using the Lashley III maze in mice. Journal of visualized experiments : JoVE, (39), 1786.
See more of Our Posts
COMPREHENSIVE BEHAVIORAL NEUROSCIENCE CATEGORY
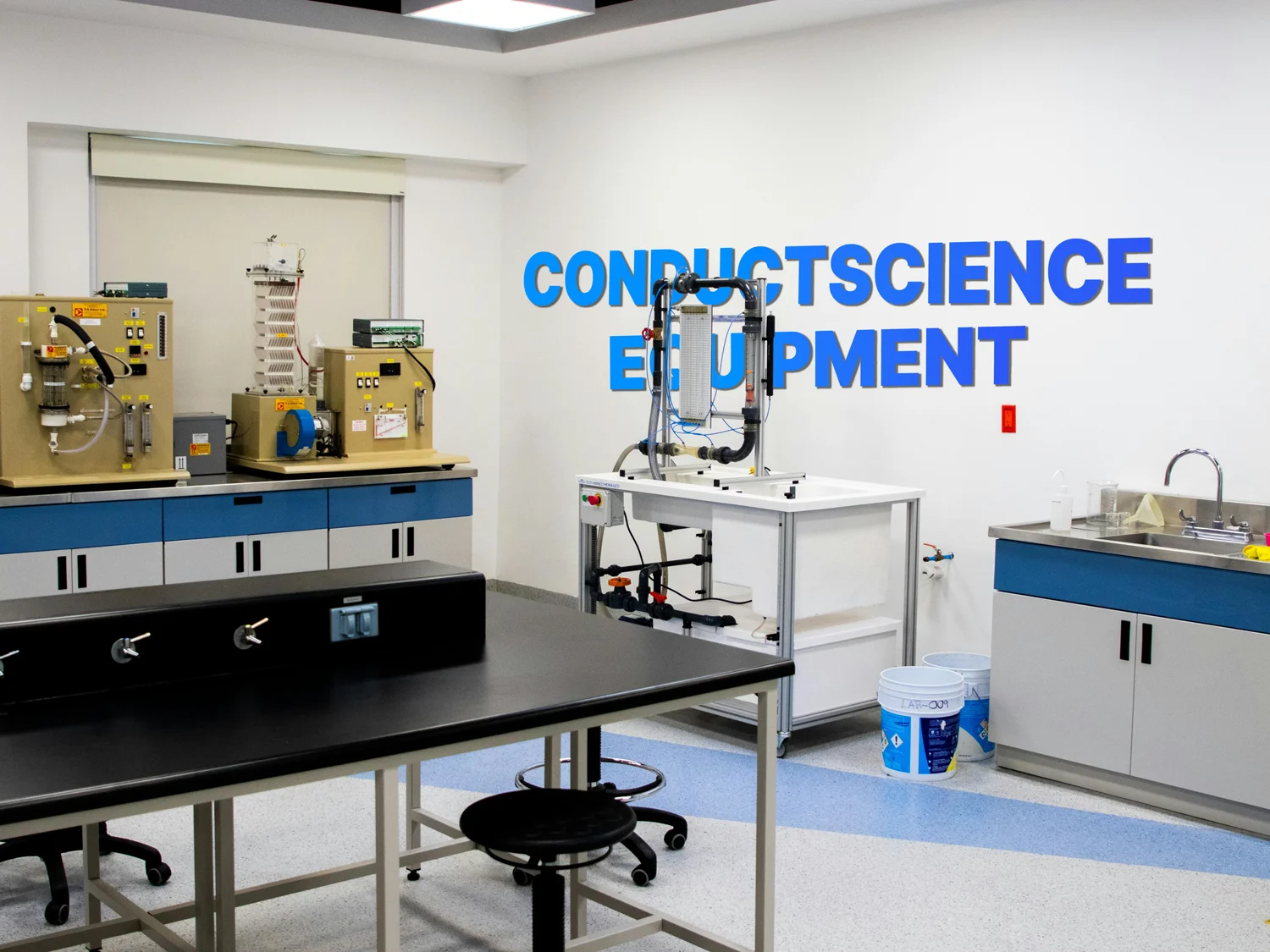
Data Management and Compliance for ConductScience Behavioral Equipment

Assessing Grip Strength in Rodents
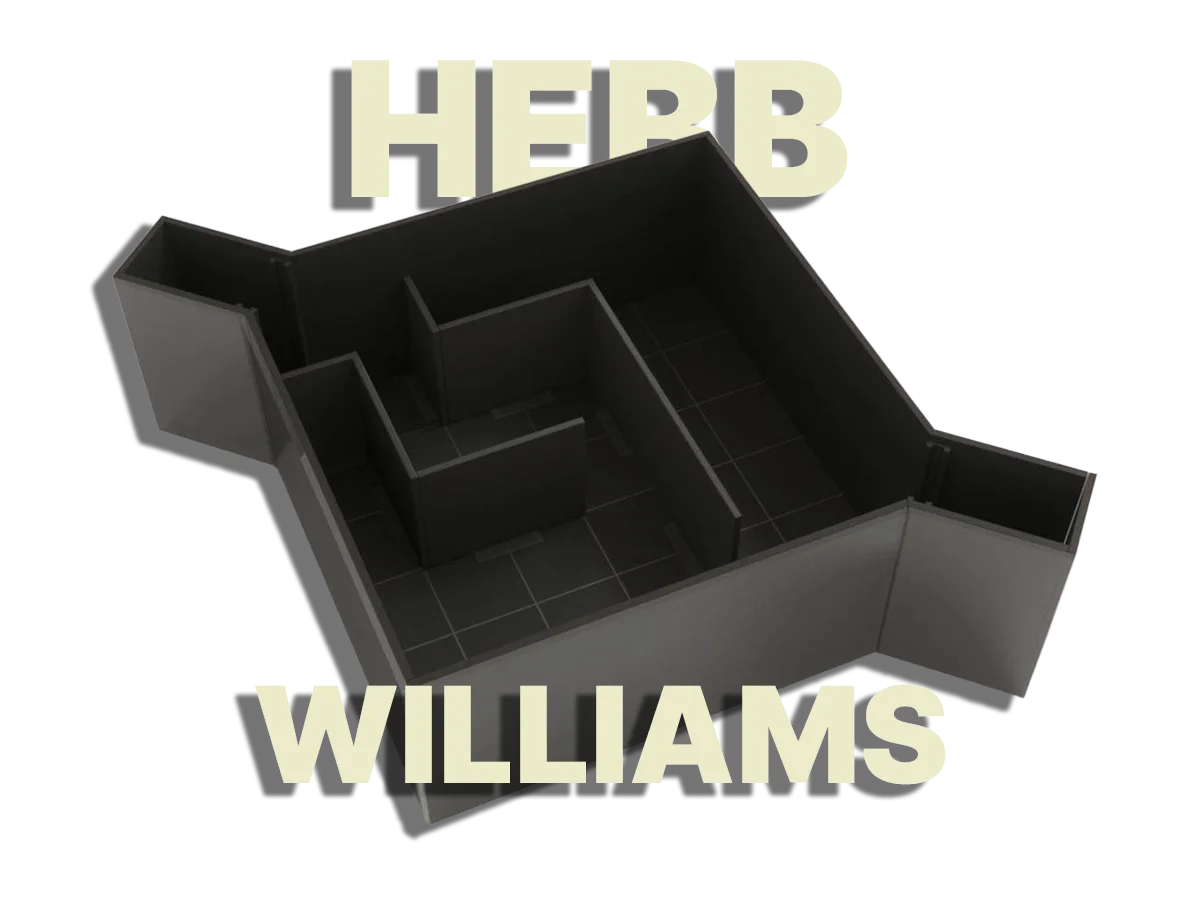
Maze Bazics: Exploring the Hebb-Williams Maze: A Tool for Cognitive Research
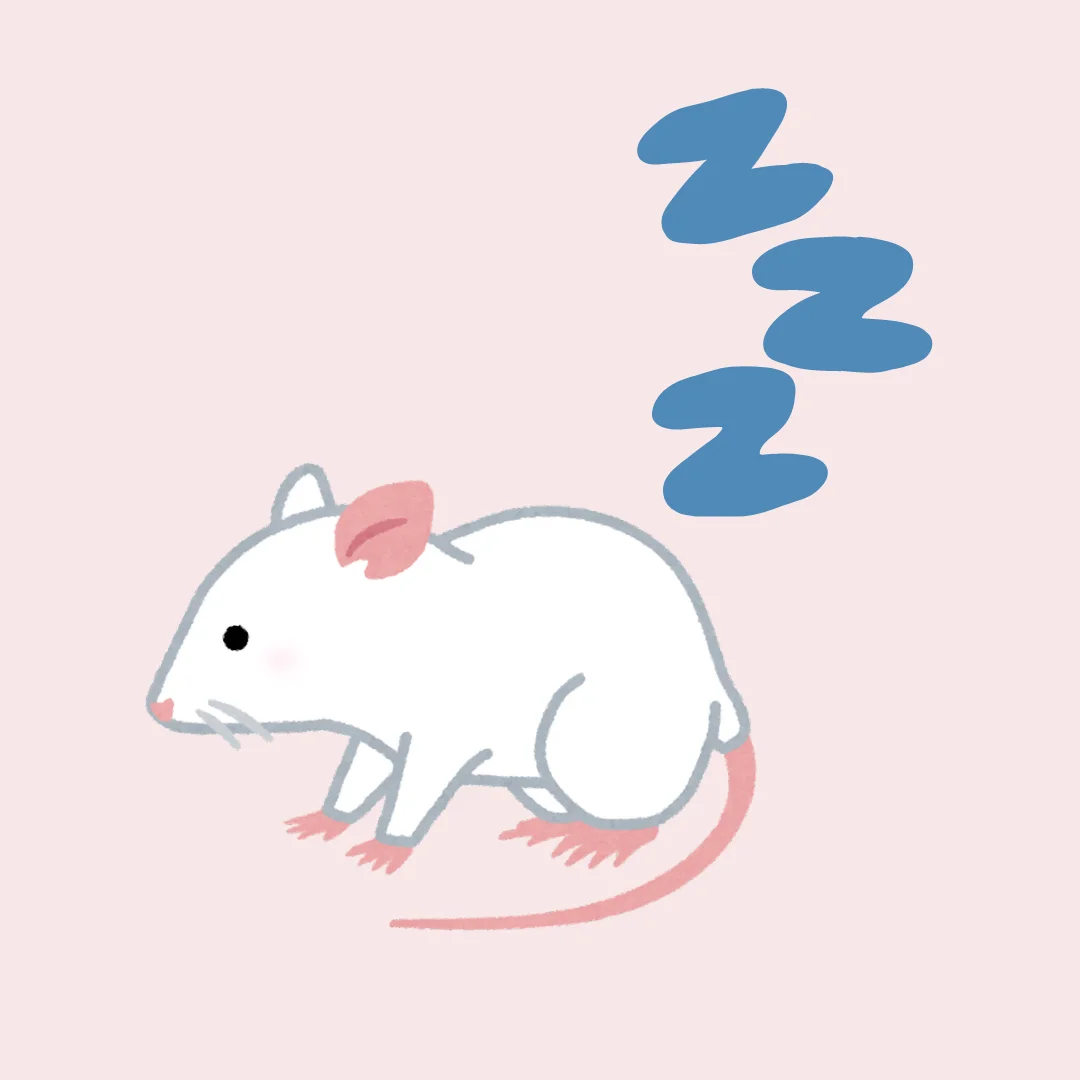
Maze Bazics: Sleep Fragmentation Chambers
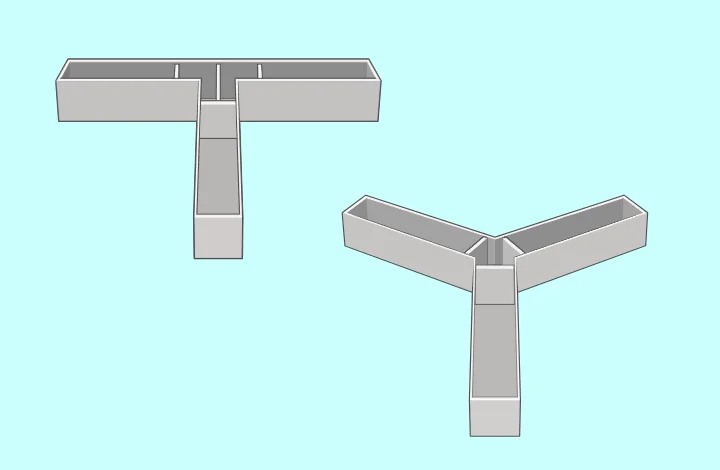
Maze Bazics: Spontaneous Alternation in Y Mazes and T Mazes
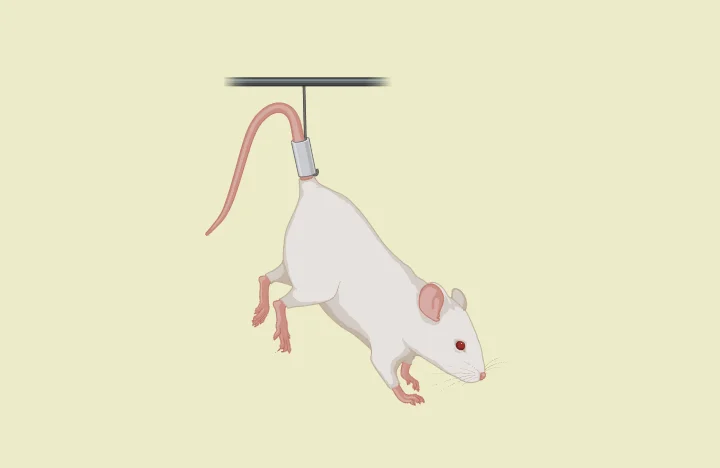
Maze Bazics: Tail Suspension Test
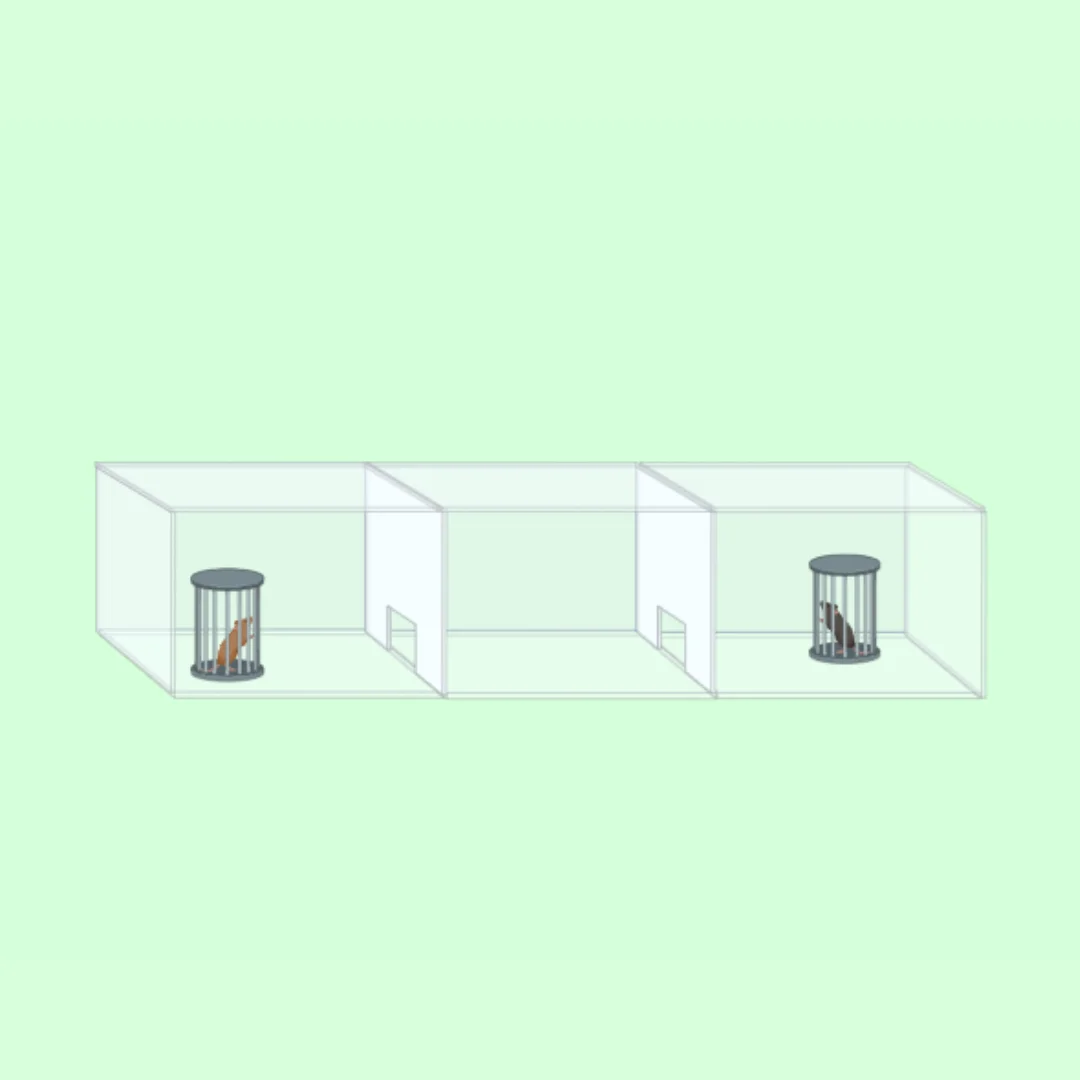
Maze Bazics: Sociability Chamber

Maze Bazics: Sucrose Preference Test
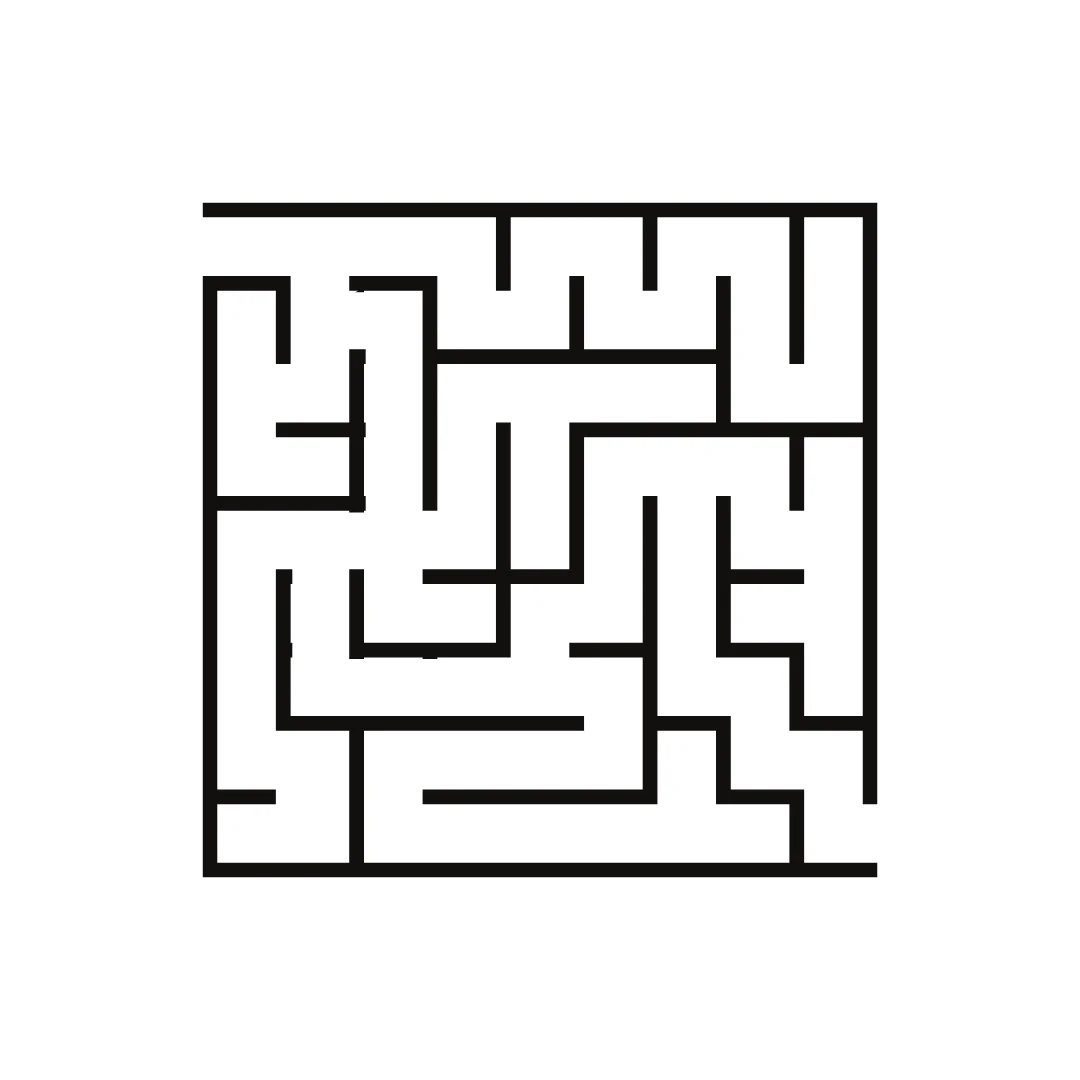
Maze Bazics: Understanding the Lashley III Maze Test
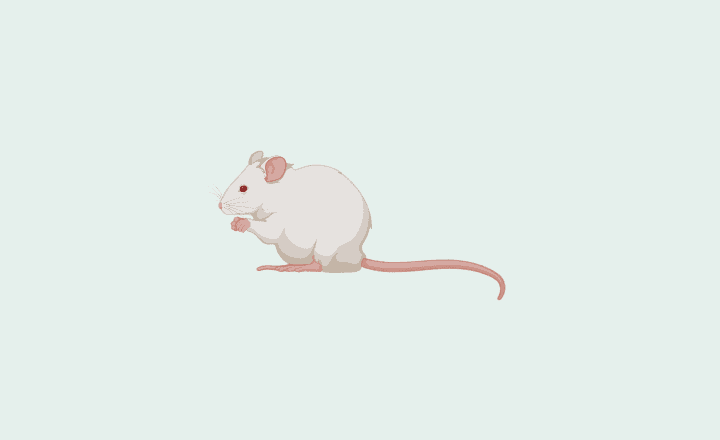
Maze Bazics: What is the Learned Helplessness Paradigm?
All Resources
Never miss our posts!
Author:
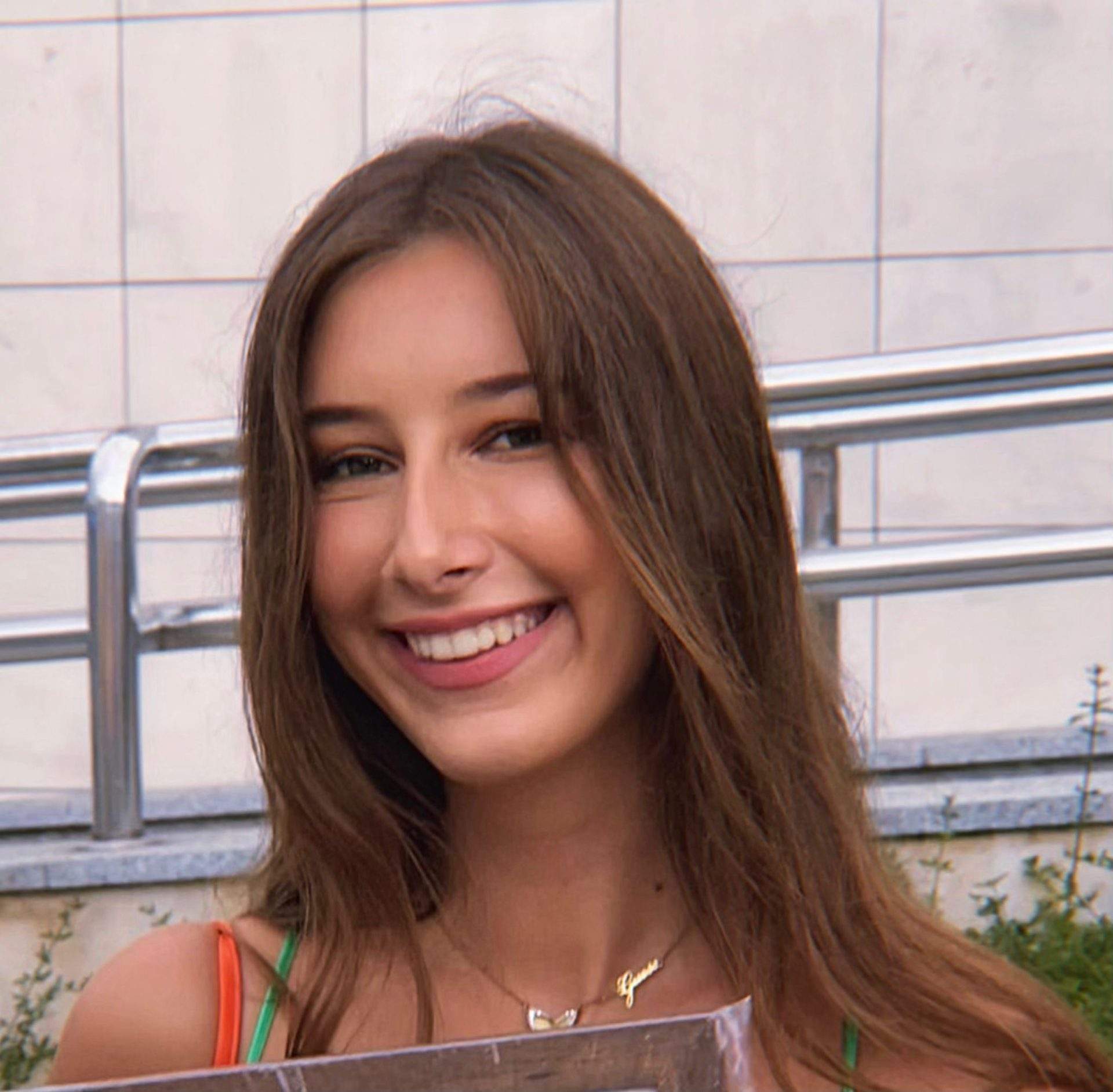
Vanja Antonijevic
Vanja works as the Social Media and Academic Program Manager at Conduct Science. With a Bachelor’s degree in Molecular Biology and Physiology and a Master’s degree in Human Molecular Biology, Vanja is dedicated to sharing scientific knowledge on social media platforms. Additionally, Vanja provides direct support to the editorial board at Conduct Science Academic Publishing House.