Reference to this article: ConductScience, What Is Optogenetics and How Does It Work? (2022). doi.org/10.55157/CS20220704
What Is Optogenetics?
Optogenetics is a method of controlling and monitoring the functions of cells, tissues, and organisms by combining specialized optical systems and genetic engineering technologies.[1]
It is now considered a branch of biotechnology because of its wide use in studying the brain’s neural circuits. Optogenetics is an evolutionary approach, without which understanding brains wouldn’t have been possible!
The technique allows researchers to turn a specific cell or cellular region on and off with precision and high resolution by using a protein called opsin. Thus, enabling them to control animals’ specific behavior (such as pain and fear) and demystifying the involvement of specific cells in controlling those behaviors.[2]
Francis Crick first proposed the concept of optogenetics in 1979 that light can be used to obtain rapid space-time dependent control over targeted neurons.[1] However, the idea wasn’t applied in neuroscience due to the unavailability of advanced tools and methods.
Optogenetics has broad applications in neurology, animal behavior, and physiology to provide insights into a spectrum of questions related to movement, sleep, blood pressure, memory, hunger, body metabolism, fear, learning, navigation, and sensory processing.[2]
This article discusses how optogenetics works, the tools and techniques required to perform optogenetic experiments, and its application to broad scientific fields.
Key Tools Involved in Optogenetics
The optogenetic experiments require three tools to perform targeted expressions and studies:
1. Opsins (or light-sensitive proteins)
Researchers require a light-activated ion channel or pump (such as opsins) to provide targeted control over electrical and biochemical events of a cell or specific regions at a particular time.[3]
Types of opsins
Opsins have the unique ability to sense and respond to light. It’s categorized into two groups based on its mode of action and primary sequence:[5]
- Type I or microbial opsins: They’re found in microorganisms such as fungi, algae, archaebacteria, and eubacteria. They include light-driven ion pumps like ion channels (e.g., channelrhodopsins ChRs, bacteriorhodopsin BRs, halorhodopsin HRs) and sensors (e.g., sensory rhodopsin I and II – SRI and SRII).[5] Microbial opsins are widely used in epigenetic research because:
- They have faster kinetics.[4]
- They are single component proteins that can easily be genetically engineered.
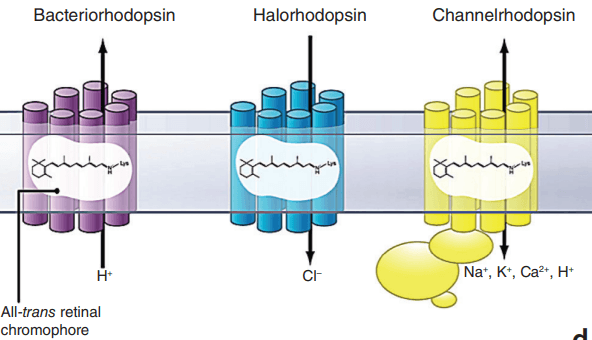
Figure: An illustrative diagram of the three main classes of microbial opsins used in single component optogenetics.[4]
- Type II or animal opsins: They are found in animals and humans; examples are rhodopsin and melanopsin. They couple with G-protein-mediated transduction pathways to mediate transmembrane ionic potential. They’re also primarily involved in circadian clocks and dim light vision.[4]
Functions of opsins
Below are different types of opsins and their purposes:[3]
- Microbial opsins like channelrhodopsins (ChR2, ChR1, VChR1, and SFOs) are used to excite neurons,[3] although anion-conducting channelrhodopsins are used for light-induced inhibition.
- Engineered potassium channels prevent the generation of action potential during blue light illumination.
- Light-driven ion pumps such as halorhodopsin (NpHR), fungal opsins (Mac), archaerhodopsin (Arch), and enhanced halorhodopsin (eNpHR2.0 and eNpHR3.0) are used to inhibit neuronal activities.[3]
- In mammals, opsins are coupled to specific G-protein coupled receptors (GPCRs) to manipulate the concentration of secondary messengers, such as cAMP and IP3, in target cells.[3]
2. Suitable plasmid or viral vector
Researchers develop direct and indirect delivery methods to deliver vectors containing genetically engineered or desired genes.
The direct delivery methods involve an electric field, laser pulse, hydrodynamic pressure, and ultrasound waves for gene delivery.[3] The indirect delivery methods include photosensitizing target regions by transplanting photosensitive cells to targeted regions.
The nanoparticles and dendrimers are also used in some experiments to deliver the protein in targeted regions. However, it has low transgene expression and delivery efficiency.
The most extensively used gene delivery methods use viral vectors such as adenoviruses, lentiviruses, and adeno-associated viruses – they offer better gene delivery efficiency than plasmid vectors.[1]
3. Light source
A high-throughput integrated fiber optic and light source are required to reach the target cells even deep within the brain. The superficial brain areas can be stimulated by directly mounting optical fibers or LEDs to the animals’ skulls. However, OLEDs (organic light-emitting diodes) are used to implant in the brain because of their thinness – less than 1 µm.[3]
Optogenetic Techniques
Three approaches are used to integrate a microbial opsin and optogenetic actuator (genetically-encoded tools to control light-activated opsin proteins) in organisms in specific regions.[3]
- 1st approach: Introduce a genetically engineered viral vector containing opsin and a suitable promoter (e.g., CAMKIIα) in the selected cell or organisms.[3] One limitation of the approach is that it’s less sensitive.
- 2nd approach: Introduce the optogenetic actuator with a suitable promoter (such as Thy1) in the transgenic mice, a mice zygote.[3] The introduction of the optogenetic actuator at an early stage allows the insertion of a larger genetic segment. Thus, providing more specificity toward integrating segments into cells than the first approach.
- 3rd approach: It’s the most efficient and modern optogenetic technique. Here, transgenic mice with Cre recombinase are created (Cre recombinase is an enzyme that catalyzes recombination between two lox-P sites).[3] Then, the genetically engineered vector with an optogenetic actuator is inserted between the lox-P site, and only cells containing the Cre recombinase can express the opsin proteins.
How Does Optogenetics Work?
The optogenetics applied today in scientific studies is composed of three core features [4]:
- Microbial opsin, a gene family of light-sensitive ion channel proteins, is found in organisms ranging from humans and algae to archaebacteria. Each gene encodes a specific protein that responds to a specific light.
- General methods for transferring opsin protein to well-defined cellular elements in the brain.
- A general method to incident light at the targeted or specific location or brain cells.[4]
In optogenetic studies, a specific genetic code of the organism is taken and attached to a protein called opsin.[6] The genetically engineered code is delivered to the targeted cell or tissues to synthesize opsin proteins in the cell. The protein glows on the incidence of a specific light, and that’s how scientists study the neuronal activities in an organism’s specific cell or region.[6]
For example, the opsin, channelrhodopsin-2 (ChR2), obtained from the green algae Chlamydomonas reinhardtii, is activated in the presence of only blue light. Thus, when the protein is delivered to the targeted neuron, it’s activated on the incidence of blue light and deactivated in its absence.[3]
Other neurons not containing opsin proteins will not be affected and will continue to function normally.
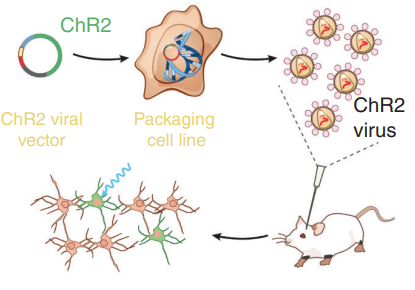
Figure: An illustrative diagram of introducing genetically altered genes with opsin protein ChR2 in a mouse via a viral vector to understand the function of a specific neuron upon incidence of blue light.[4]
Applications Of Optogenetics
Optogenetics has several applications in life sciences, especially neuroscience and neurobiology, where it helps us understand neural circuitry or brain activities underlying diseases and behavior.[1] The technique has replaced untargeted, highly invasive, and slow-kinetic approaches to studying neurons.[1]
Have a look at how the optogenetic technology is involved in the deciphering factors underlying disease conditions, such as Huntington’s disease and Schizophrenia:[3]
- Researchers have used optogenetics to map the neural circuit in the amygdala — a part of the brain that controls fear. In this process, Channelrhodopsin-2 attached with a parvalbumin-Cre promoter was introduced in the transgenic mice. After optically stimulating the neurons with light, freezing behavior was observed in the mice at 4 Hz, which confirmed the amygdala’s involvement in fear response at the given frequency.[3]
- The optogenetic technique has contributed to understanding olfactory sensory neurons for demonstrating timing in odor processing.[3] Moreover, it’s also used to understand the mechanism of neuromodulatory mediated olfactory guided behaviors and precise brain regions where the odor is more concentrated.
- Researchers have recently found that optical interrogation of developing zebrafish with ChR2- and NpHR- expressing hearts can be better used to map the time-dependent development and overlap of cardiac conduction systems.[1]
- Optogenetics is applied in mapping cardiac biological functions, such as calcium and transmembrane voltage changes. It provides insight into the heart’s physiological responses and processes during heart development, homeostasis, and diseases.[1]
- Researchers have used optogenetics to restore the auditory activity of a deaf mouse by stimulating its spiral ganglion.[3]
- The potential of optogenetic technology in activating or inhibiting neurons has been used to understand a range of motor behavior, including interneurons’ activity in altering locomotion, localizing tactile sensory input, and functions of Purkinje cells in generating and modulating movement.[3]
Conclusion
Optogenetic is a tool used to understand the specific neuronal function contributing to an organism’s physiology and behavior, such as fear, fight, hunger, and disease conditions, by combining optical technologies and genetic engineering.
It helps map the neural circuitry and provides deeper insight into the role of a brain region or specific neuron in regulating certain behavior or disease conditions by using a protein called opsin. The optogenetic technology has extensive use in neurobiology, cardiology, physiology, and behavioral studies of organisms.
Its use is still developing in many clinical areas, including cardiac and therapeutic conditions. Therefore, current research centers on increasing the efficacy of optogenetic stimulation in clinics.
The development of opsins with increased photosensitivity, suitable light-delivery devices, and bigger model systems (like a pig) will enable the technology to unravel many mysteries related to our brains.
Check out our complete optogenetic system and accessories to assist your experiment if you need a high-quality optogenetic machine.
References:
- Joshi J, Rubart M and Zhu W (2020) Optogenetics: Background, Methodological Advances and Potential Applications for Cardiovascular Research and Medicine. Front. Bioeng. Biotechnol. 7:466. DOI: 10.3389/fbioe.2019.00466.
- Deisseroth Karl. Optogenetics. Retrieved from https://www.britannica.com/science/optogenetics
- Optogenetics. Retrieved from https://en.wikipedia.org/wiki/Optogenetics#cite_note-Johansen_2010-102
- Deisseroth, K. (2015). Optogenetics: 10 years of microbial opsins in neuroscience. Nature Neuroscience, 18(9), 1213–1225. doi:10.1038/nn.4091
- Duebel, J., Marazova, K., & Sahel, J. A. (2015). Optogenetics. Current opinion in ophthalmology, 26(3), 226–232. https://doi.org/10.1097/ICU.0000000000000140
- Lim D and LeDue J (2017) What Is Optogenetics and How Can We Use It to Discover More About the Brain?. Front. Young Minds. 5:51. DOI: 10.3389/frym.2017.00051