Introduction
Neuroscience Research:
- Brain Mapping: Researchers use the stereotaxic apparatus to map brain functions by precisely targeting and manipulating specific areas. This helps in understanding the roles of different brain regions in behavior, cognition, and sensory processing [2].
- Neurotransmitter Studies: The apparatus enables the accurate injection of chemicals, allowing the study of neurotransmitter systems. Techniques such as microdialysis can be employed to measure neurotransmitter release and uptake in specific brain regions [3].
- Electrophysiology: It facilitates the placement of electrodes to record electrical activity from neurons, providing insights into the neural basis of behavior and disease [4].
Pharmacological Research:
- Drug Delivery: Precisely delivers pharmacological agents directly into targeted brain areas, allowing for the study of drug effects on specific neural circuits [5].
- Gene Therapy: The apparatus can deliver viral vectors carrying genetic material to specific brain regions, aiding in gene therapy research for neurological disorders [6].
Behavioral Studies:
- Lesion Studies: By creating controlled lesions in specific brain areas, researchers can study the resulting behavioral changes, contributing to our understanding of brain function and dysfunction [7].
- Implantation of Devices: Chronic implantation of devices such as cannulas or optical fibers allows for long-term manipulation and observation of neural activity during behavioral experiments [8].
Advanced Neuroscience Techniques:
- Optogenetics: This technique involves the insertion of light-sensitive proteins into neurons, which can then be controlled with light. The stereotaxic apparatus ensures the precise placement of optical fibers for light delivery [9].
- Fiber Photometry: It allows real-time monitoring of neural activity by measuring fluorescent signals from genetically encoded indicators. Precise placement of optical fibers is crucial for accurate data collection [10].
- Two-Photon Imaging: For high-resolution imaging of neuronal activity, the apparatus is used to stabilize and target the imaging equipment to specific brain regions [11].
Clinical Applications:
- Deep Brain Stimulation (DBS): In the clinical setting, the stereotaxic apparatus is essential for the precise placement of electrodes in the treatment of conditions like Parkinson’s disease, essential tremor, and dystonia [12].
- Biopsies: Stereotaxic apparatus allows for the accurate collection of brain tissue samples for diagnostic purposes, minimizing damage to surrounding healthy tissue [13].
Developmental Studies:
Embryonic and Neonatal Research: The apparatus can be adapted for use with very small animal subjects, such as embryos or neonates, facilitating studies on brain development and the effects of early-life interventions [14].
Parkinson’s Disease
The stereotaxic apparatus is frequently utilized in Parkinson’s disease research, as demonstrated in a recent study by Landeck et al. (2021).
Parkinson’s disease (PD) is a progressive disorder traditionally defined by resting tremor and akinesia, primarily due to the loss of dopaminergic neurons in the substantia nigra. Studies using stereotaxic injections deliver chemicals, proteins, or viral vectors to mimic various aspects of PD [15].
By injecting 6-OHDA (oxidopamine) into the brain, researchers caused a major loss of dopaminergic neurons, resulting in significant motor deficits. 6-OHDA is taken up by monoamine transporters and blocks mitochondrial respiration [15].
By destroying nigral dopaminergic neurons, the primary symptoms of Parkinson’s disease can be replicated, making rats used in stereotaxic surgery an effective model for Parkinson’s disease research.
Depression Models
Stereotaxic surgery is also utilized to create animal models of depression, which are essential for studying the underlying mechanisms and testing potential treatments for the disorder. Here’s how it can be done:
- Lesioning Specific Brain Regions: By using stereotaxic surgery to precisely lesion specific brain areas, such as the prefrontal cortex or hippocampus, researchers can induce behaviors in animals that resemble depressive symptoms. These brain regions are known to be involved in mood regulation, and their disruption can mimic aspects of depression [16].
- Chronic Stress Models: Implanting chronic stress devices, such as osmotic minipumps, allows for the continuous delivery of stress hormones like corticosterone. This can simulate chronic stress conditions in animals, leading to depressive-like behaviors. Stereotaxic surgery ensures these devices are accurately placed to produce consistent and reliable results [17].
- Viral Vector Delivery: Stereotaxic surgery can be used to deliver viral vectors carrying specific genes or RNA sequences into targeted brain regions. For instance, overexpressing or knocking down genes implicated in depression (like BDNF or serotonin receptors) can help create a genetic model of depression. This precise delivery alters the expression of these genes, resulting in depressive-like phenotypes [18].
- Pharmacological Models: Direct infusion of pharmacological agents into specific brain areas using stereotaxic surgery can also create depression models. For example, infusing glutamate receptor antagonists into the prefrontal cortex can produce symptoms resembling those of depression, allowing for the study of glutamatergic dysfunction in mood disorders [19].
- Optogenetics and Chemogenetics: Advanced techniques like optogenetics and chemogenetics involve using stereotaxic surgery to implant optical fibers or designer receptors in specific brain regions. These techniques enable precise control over neuronal activity, allowing researchers to induce or inhibit depressive-like behaviors by modulating neural circuits in real-time [20].
Conclusion
The stereotaxic apparatus is a key tool in modern neuroscience and biomedical research, allowing for precise targeting of specific brain regions. It is used in a variety of applications, including basic neuroscience research where it helps map the brain and study neurotransmitter systems, as well as in behavioral studies that create controlled lesions or involve the long-term implantation of devices for observation. In pharmacological research, the apparatus enables the accurate delivery of drugs and gene therapy vectors to specific brain areas, which is crucial for understanding their effects on neural circuits. It is also essential for advanced techniques like optogenetics, fiber photometry, and two-photon imaging, ensuring the correct placement of equipment needed for these methods. Clinically, the stereotaxic apparatus is used in procedures such as deep brain stimulation for Parkinson’s disease, where precise electrode placement is necessary for effective treatment. Additionally, it can be adapted for use in developmental studies with embryos or neonates, providing insights into brain development and early-life interventions. Overall, the stereotaxic apparatus is a vital tool that advances our understanding of the brain and aids in developing new treatments for neurological disorders.
References
- Yang, P., Wang, Z., Zhang, Z., Liu, D., Manolios, E. N., Chen, C., Yan, X., Zuo, W., & Chen, N. (2018). The extended application of The Rat Brain in Stereotaxic Coordinates in rats of various body weight. Journal of neuroscience methods, 307, 60–69. https://doi.org/10.1016/j.jneumeth.2018.06.026
- Paxinos, G., & Watson, C. (2007). The rat brain in stereotaxic coordinates: Hard cover edition. Elsevier.
- Benveniste, H., & Hansen, A. J. (1990). Microdialysis: Theory and application. Progress in Neurobiology, 35(3), 195-215.
- Buzsáki, G. (2004). Large-scale recording of neuronal ensembles. Nature Neuroscience, 7(5), 446-451.
- Paxinos, G., & Watson, C. (1998). The rat brain in stereotaxic coordinates (4th ed.). Academic Press.
- Davidson, B. L., & Breakefield, X. O. (2003). Viral vectors for gene delivery to the nervous system. Nature Reviews Neuroscience, 4(5), 353-364.
- Kandel, E. R., Schwartz, J. H., & Jessell, T. M. (2000). Principles of Neural Science (4th ed.). McGraw-Hill.
- Paxinos, G., & Watson, C. (2005). The mouse brain in stereotaxic coordinates. Elsevier.
- Deisseroth, K. (2011). Optogenetics. Nature Methods, 8(1), 26-29.
- Cui, G., Jun, S. B., Jin, X., Luo, G., Pham, M. D., Lovinger, D. M., & Costa, R. M. (2013). Deep brain optical measurements of cell type–specific neural activity in behaving mice. Nature Protocols, 8(8), 1609-1621.
- Helmchen, F., & Denk, W. (2005). Deep tissue two-photon microscopy. Nature Methods, 2(12), 932-940.
- Benabid, A. L., Pollak, P., Gao, D., Hoffmann, D., Limousin, P., Gay, E., & Perret, J. (1996). Chronic electrical stimulation of the ventralis intermedius nucleus of the thalamus as a treatment of movement disorders. Journal of Neurosurgery, 84(2), 203-214.
- Apuzzo, M. L., & Heiden, J. S. (1984). The use of computed tomography in stereotactic surgery. Acta Neurochirurgica, 70(1), 21-31.
- Paxinos, G., & Franklin, K. B. J. (2001). The mouse brain in stereotaxic coordinates (2nd ed.). Academic Press.
- Landeck N, Conti Mazza M, Duffy M, Bishop C, Sortwell CE, Cookson MR. Stereotaxic Intracranial Delivery of Chemicals, Proteins or Viral Vectors to Study Parkinson’s Disease. J Vis Exp. 2021 Feb 18;(168):10.3791/62128. doi: 10.3791/62128. PMID: 33682858; PMCID: PMC8981414.
- Nestler, E. J., & Hyman, S. E. (2010). Animal models of neuropsychiatric disorders. Nature Neuroscience, 13(10), 1161-1169.
- Willner, P. (2005). Chronic mild stress (CMS) revisited: consistency and behavioural-neurobiological concordance in the effects of CMS. Neuropsychobiology, 52(2), 90-110.
- Berton, O., & Nestler, E. J. (2006). New approaches to antidepressant drug discovery: beyond monoamines. Nature Reviews Neuroscience, 7(2), 137-151.
- Krystal, J. H., Sanacora, G., & Duman, R. S. (2013). Rapid-acting glutamatergic antidepressants: the path to ketamine and beyond. Biological Psychiatry, 73(12), 1133-1141.
- Tye, K. M., & Deisseroth, K. (2012). Optogenetic investigation of neural circuits underlying brain disease in animal models. Nature Reviews Neuroscience, 13(4), 251-266.
See more of Our Posts
stereotaxic on resources
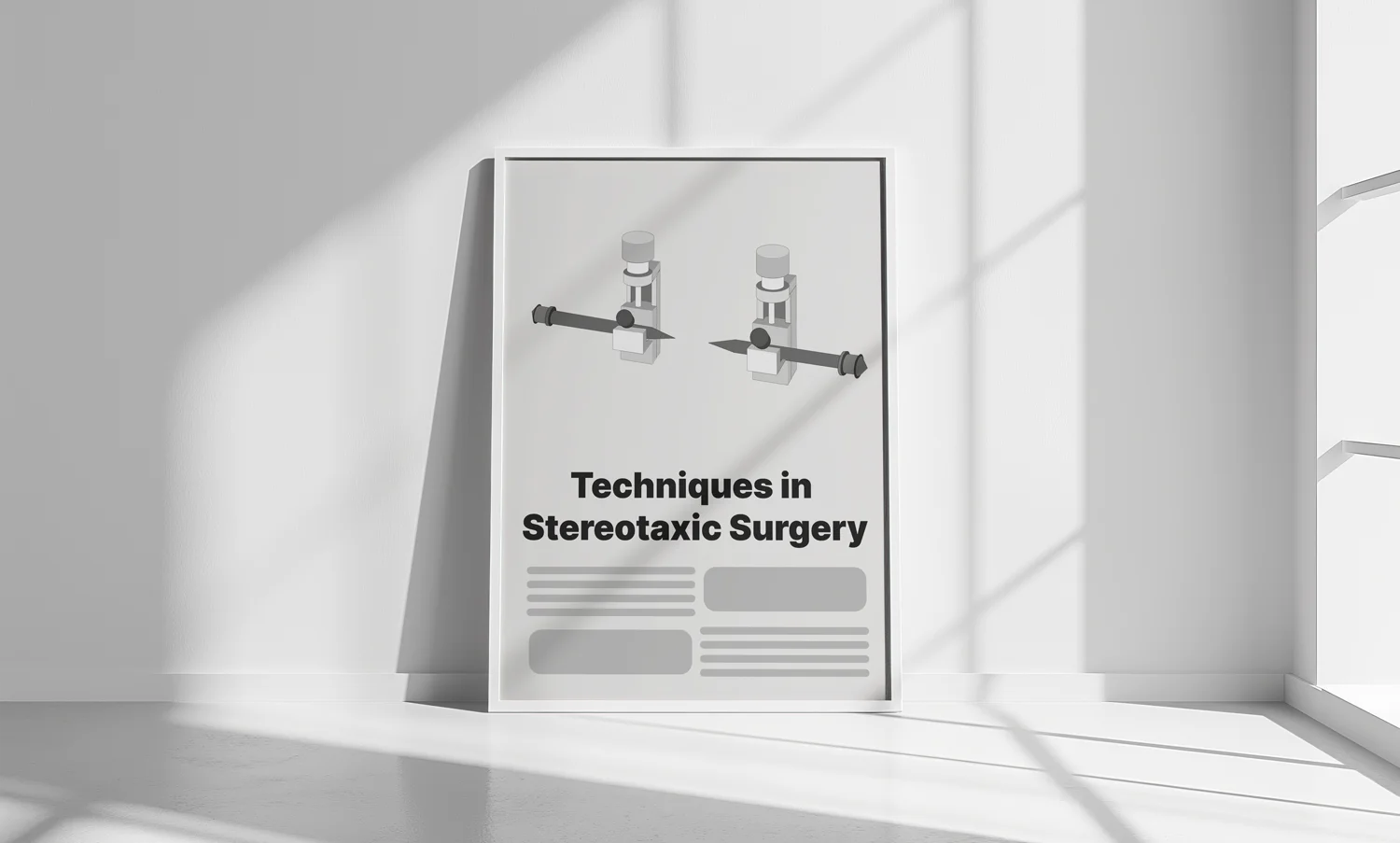
Techniques in Stereotaxic Surgery
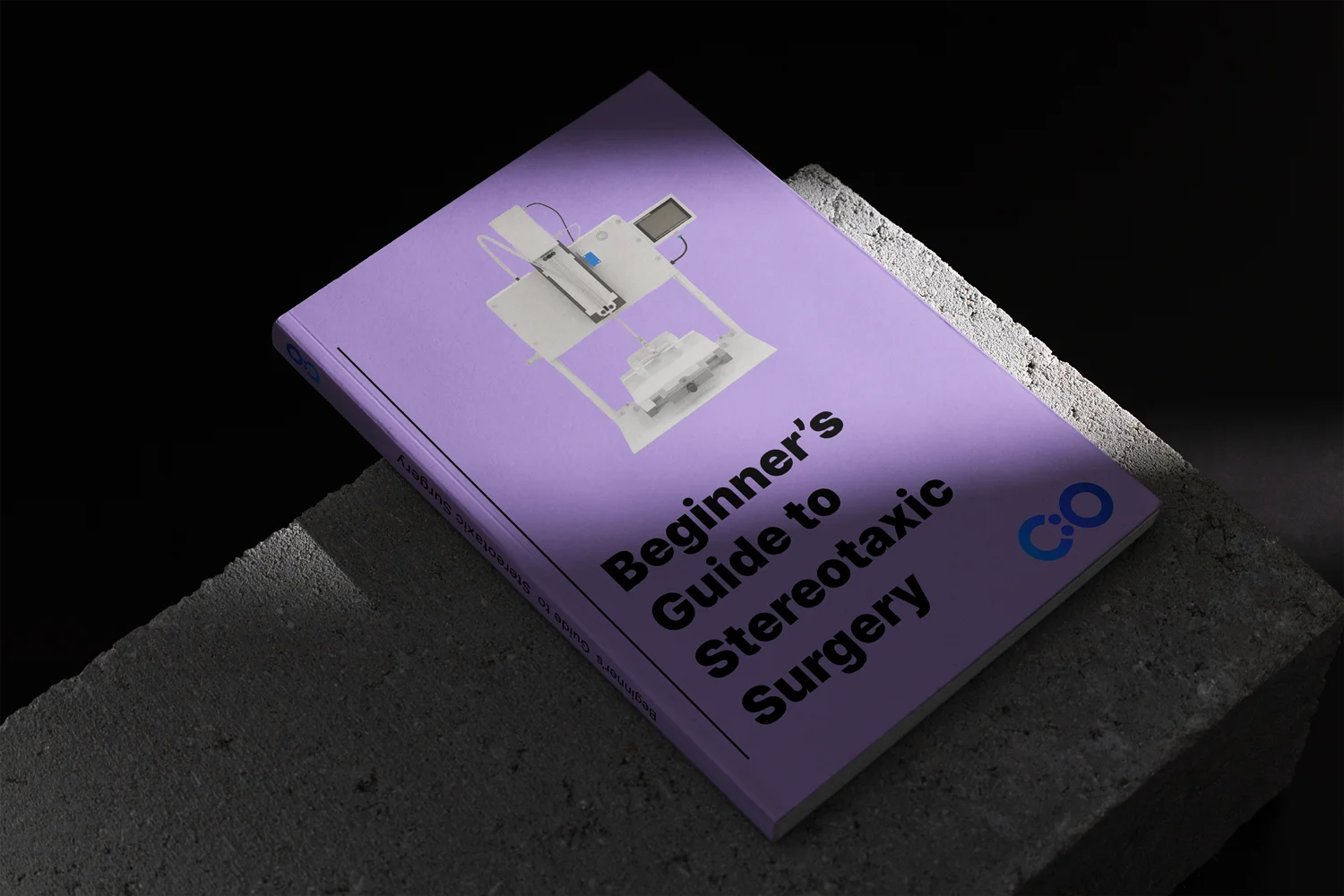
Beginner’s Guide to Stereotaxic Surgery
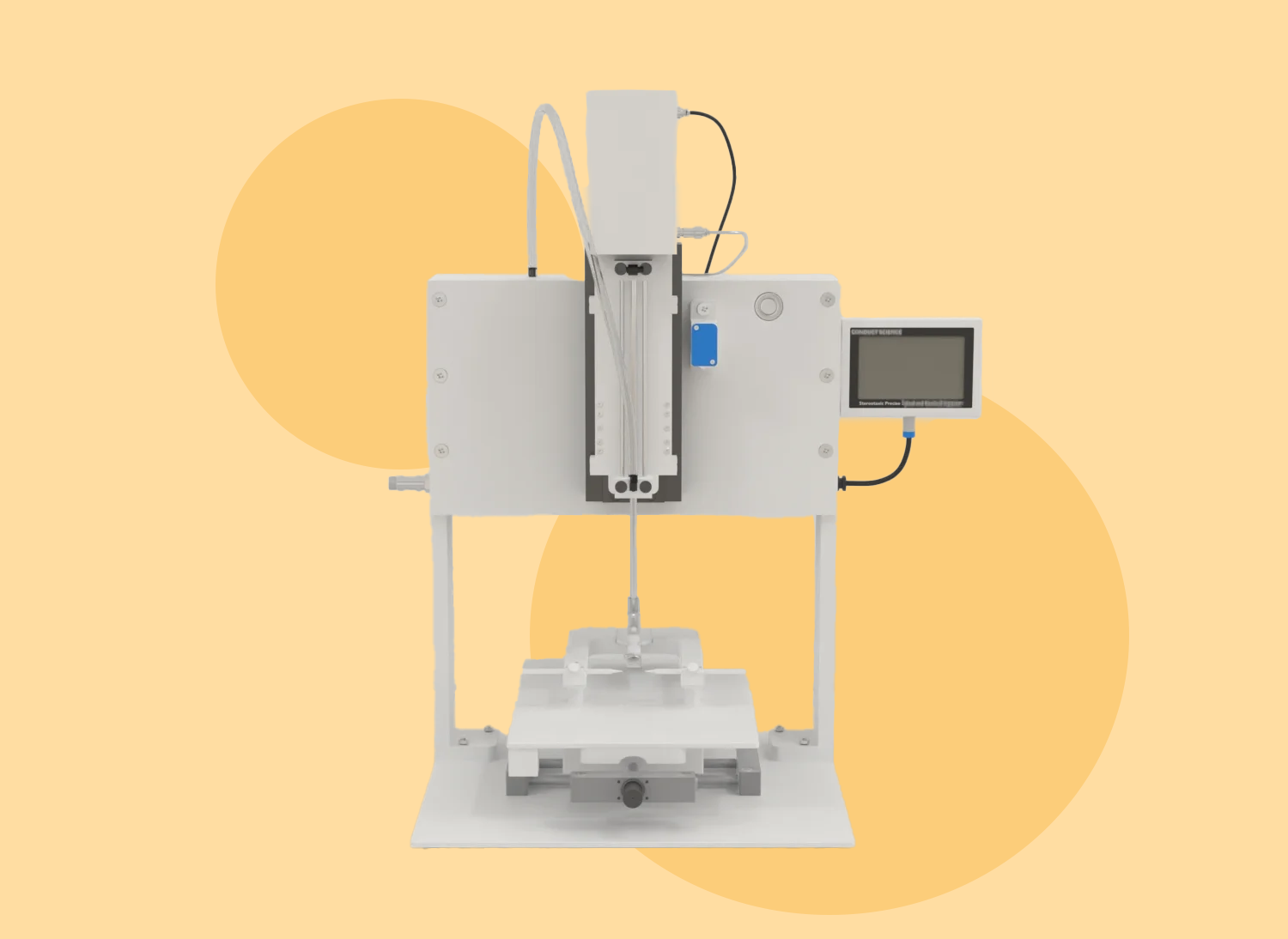
Stereotaxic Impactor for Traumatic Brain Injury (TBI) Induction
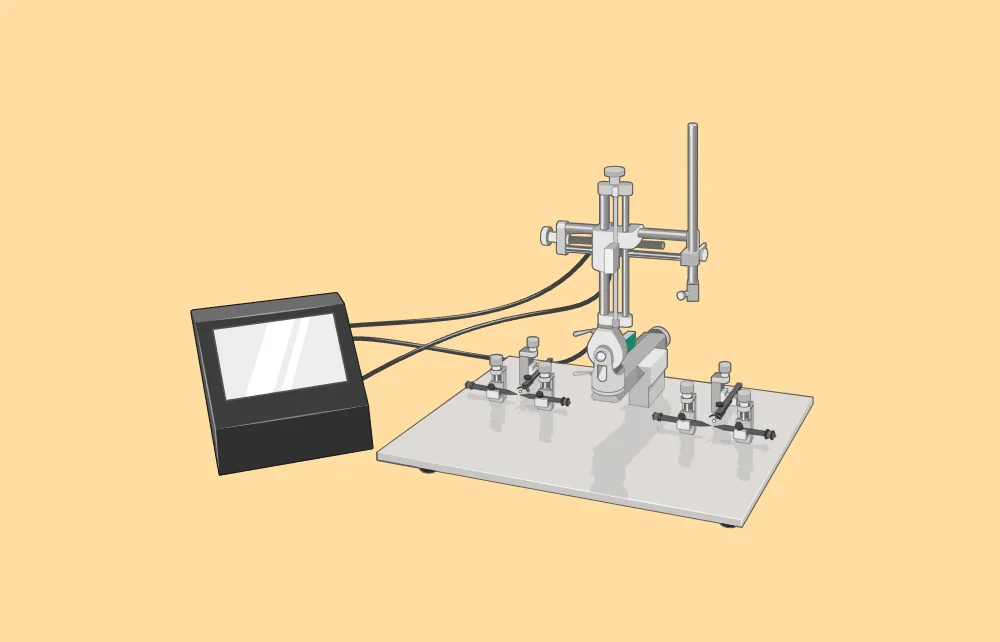
Applications of the Stereotaxic Apparatus
All Resources
Never miss our posts!
Author:

Louise Corscadden, PhD
Dr Louise Corscadden acts as Conduct Science’s Director of Science and Development and Academic Technology Transfer. Her background is in genetics, microbiology, neuroscience, and climate chemistry.