Introduction
Medical advancements are an essential requirement in today’s world to reduce the financial burden of diseases and safeguard the healthcare systems. For diseases like cancer, better drug delivery approaches, diagnostic methods, and treatment options are required to save more patients and enhance their quality of life.
One such approach developed — being tested, and applied in some disease cases — is the use of liposomes to enhance drug delivery in organisms. And besides drug delivery, they also have applications in analytical biochemistry and medical diagnostics.
Liposomes are little bubbles (vesicles) made of the same substances as cell membranes. They get their names from two Greek words: ‘Lipos,’ which means fat, and ‘Soma,’ meaning body.[1]
They were discovered in 1964 by a British hematologist Dr. Alec D Bangham, and another scientist, R. W. Horne. They were discovered by applying negative stains to dry phospholipids when the two scientists tested the institute’s new electron microscope.[1]
In this article, you will learn more about liposomes: what they are, their structural composition, how they are formed, their classification, properties, advantages and disadvantages, and their applications in biomedicine/pharma to develop better diagnostic and treatment approaches.
Liposomes and Their Structural Composition
Liposomes are circular-shaped vesicles formed by one or more biological bilayer membranes that separate aqueous fluids.[2] They have two main parts: hydrophilic polar head groups that can either be zwitterionic (having both positive and negative charges) or negatively charged; and hydrophobic hydrocarbon chains with different chain lengths and degrees of unsaturation.[2]
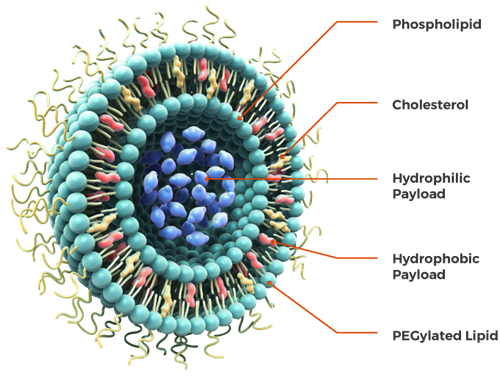
Figure: An illustration of liposome and its structural components.[3]
Liposomes structurally consist of cholesterol, and non-toxic phospholipids, explained below in detail.[2]
Phospholipids
- Glycerophospholipids are the most common phospholipids found in eukaryotic cells, with glycerol serving as the backbone. They have a hydrophilic head group and a hydrophobic side chain in their chemical structure.[2]
Further variation in the head groups results in different glycerophospholipids, such as phosphatidylcholine, phosphatidylethanolamine, phosphatidylserine, phosphatidylinositol, phosphatidic acid, phosphatidylglycerol, and cardiolipin.[2]
- Sphingomyelins are another critical component of the biological membrane. They consist of a backbone, sphingosine, and have cis-double bonds in each of their molecules connected through amide bonds in the acyl chains. They can form both intermolecular and intramolecular hydrogen bonds.[2]
Cholesterol
Cholesterol alone can’t form the liposome structure. However, with phospholipids, cholesterol can be incorporated into liposomes, improving the stability of the vesicle and preventing the aggregation of molecules. It’s also an essential factor involved in the regulation of the fluidity of liposomes.[4]
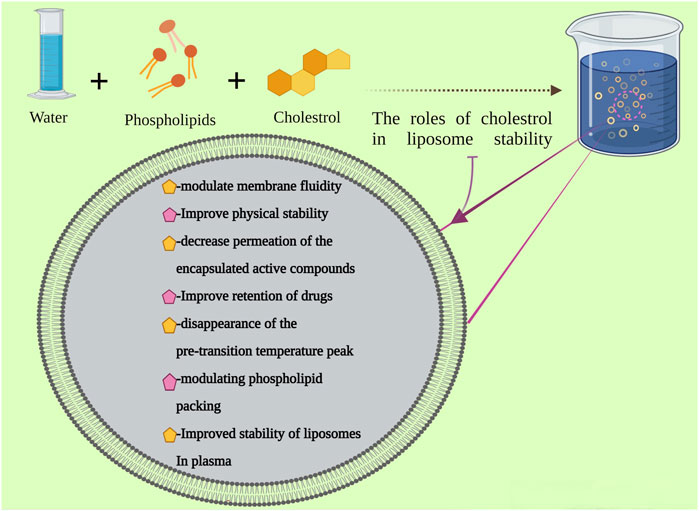
Figure: An illustration of the preparation of liposomes and cholesterol roles in its stability.[5]
Other Molecules
Besides phospholipids and cholesterol, some other additives are added while preparing synthetic or semisynthetic liposomes.
The presence of polyethylene glycol (PEG) on the surface of the liposomes can protect the metabolic inactivation and degradation of combined drugs, increase the circulation time of liposomes systemic circulation, and enhance the intracellular uptake of liposome vesicles.[4]
For the preparation of charged liposomes, charged phospholipids, like stearyl amine (SA) and dicetyl phosphate (DCP), are used.[4]
Classification of Liposomes
Liposomes are versatile molecules and can be classified in several ways based on their diversity and structural properties, such as composition, shape, size, and surface properties.
1. Based on their size, they are classified as:
- Unilamellar liposomes: They are spherical vesicles confined by a single bilayer of an amphiphilic lipid or a combination of such lipids. And they contain an aqueous solution in the center of the vesicle.
- Multilamellar liposomes: They have an onion-like structure and are made by numerous unilamellar vesicles, forming on the inside of other unilamellar vesicles with smaller sizes. Thus, it forms a multilamellar structure of concentric phospholipid spheres separated by water layers.
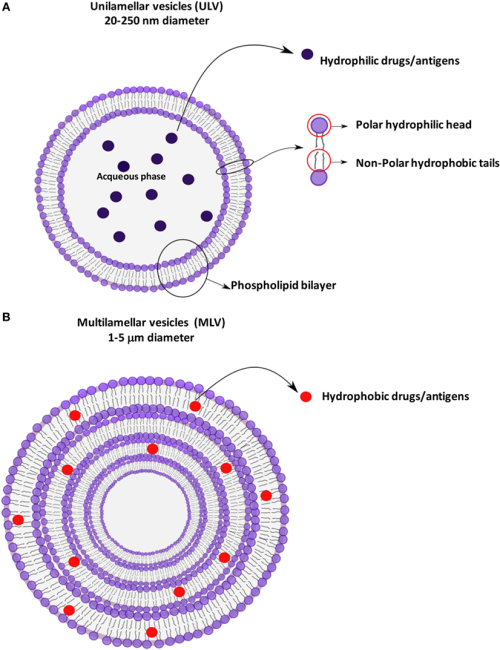
Figure: An illustration of unilamellar and multilamellar vesicular structures.[6]
2. Based on their structural parameters, they are categorized into:
- Multilamellar liposomes/vesicles (MLV): They consist of more than five multiple lipid bilayers, forming a multilamellar vesicle.
- Oligolamellar vesicles (OLV): Vesicles containing concentric lamellae, ranging between two to five, are called oligolamellar vesicles.
- Multivesicular liposomes/vesicles (MVV): They are also known as vesosomes—meaning “vesicles-inside-vesicles.”[7] In an aqueous solution, they contain internal, non-concentrically arranged smaller vesicles made from naturally occurring or synthetic bilayer-forming amphiphiles.[7]
- Unilamellar vesicles (ULV): It consists of a single lipid bilayer further divided into giant unilamellar liposomes/vesicles (GUV) groups, large unilamellar liposomes/vesicles (LUV), medium unilamellar vesicles (MUL), and small unilamellar liposomes/vesicles (SUV).

3. Liposomes are synthesized using an extensive range of methods. Based on synthesis, they are classified as:
- Dehydration and rehydration (DRV): In this process, the small unilamellar vesicles containing the buffer are dried and rehydrated with the aqueous solution, containing the compound needed to be incorporated in the vesicle.[2] This method is generally used to form an oligolamellar vesicle.
- Reverse phase evaporation (REV): This technique is based on the formation of inverted micelles. They are formed after sonicating a mixture of a buffered aqueous phase (containing the water-soluble molecules to be encapsulated into the liposomes) and an organic phase in which the amphiphilic molecules are solubilized.[8]
- Extrusion technique (VET): Here, multilamellar vesicles are pushed through a polycarbonate membrane filter containing pores of the desired size to control the vesicle size distribution.[2]
- Freeze and thaw extrusion method (FAT): In this method, liposomes formed using the thin-film method are vortexed with the material needed to be incorporated in the vesicles. This is done until the whole lipid film is suspended. Then, the resulting vesicles are frozen in warm water and vortexed again.[2]
- Sonication: It’s one of the most extensively used techniques for liposome preparation. Here, multilamellar vesicles formed by other preparation techniques are subjected to sonication, resulting in small unilamellar vesicles.[2]
- Solvent dispersion method: It includes ether injection and ethanol injection methods.
- Ether injection method: Here, a lipid solution mixed with diethyl ether or an ether-methanol mixture is progressively injected into an aqueous solution, containing the substance to be encapsulated, at 55°C to 65°C or under reduced pressure. Then, ether is eliminated under a vacuum that leads to the formation of liposomes.[8]
- Ethanol injection method: Here, a lipid solution dissolved in ethanol is rapidly injected into a large buffer volume, resulting in the rapid formation of multilamellar liposomes/vesicles.[8]
- Thin-film hydration method: This is the most common and widely used technique. In this method, the lipids are dissolved in an organic solvent, like chloroform or mixtures of chloroform and methanol.[2] Then, the solvent is removed by film deposition under vacuum.
- Conventional liposomes: They are the first liposomes created and used in pharmaceutical applications. They consist of natural lipids or phospholipids, like 1, 2-distearoyl-sn-glycero-3-phosphatidylcholine (DSPC), sphingomyelin, egg phosphatidylcholine, and monosialoganglioside.[1]
- pH-sensitive liposomes: Their design is inspired by viruses that merge with endosomal membranes and deliver their genetic material to the cytosol before reaching the lysosomes.[5] They generally consist of phosphatidylethanolamine and titratable stabilizing amphiphilic molecules that are unstable under acidic conditions.[5]
- Cationic liposomes: They are formed by mixing the cationic lipids, like 1,2-di-O-octadecenyl-3-trimethylammonium propane (DOTMA), with DNA. The complex form is due to the interaction between the positive head group of the lipid with the negative phosphate group of DNA.[1]
- Immune liposomes: These liposomes contain monoclonal antibodies or their fragments with phospholipids. In some cases, the antigens are reconstituted into liposomes membrane or inserted into the interior core of the liposome to enhance immune response.[1]
- Long circulating liposomes: These are designed to extend the circulation time of liposomes in blood. Maximum circulation time is achieved by using polyethylene glycol covalently bound to the phospholipid. They are a perfect fit to improve tissue localization.[1]
4. Liposomes can also be classified depending on their composition as:
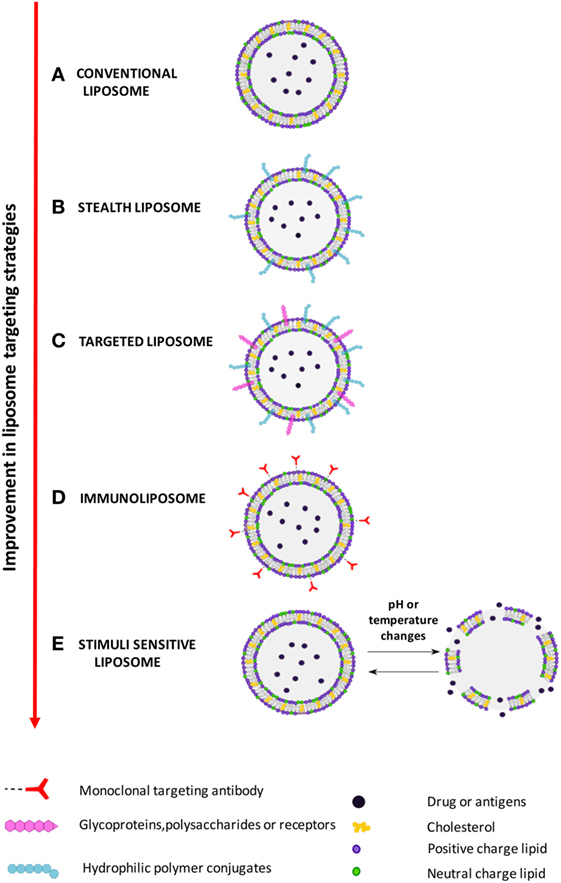
Figure: An illustration of different types of liposomes based on their composition and targeting strategies.[6]
Properties of Liposomes
Some of the properties of liposomes imparted due to their structural characterization and organization are:[1]
- They are amphiphilic.
- They are permeable to water.[1]
- Osmotically, they are sensitive.
- The liposomes with positively charged membranes are impermeable to cations, and those containing negative charges are relatively permeable to anions.[1]
- The extensive sizes range of liposomes are:[9]

Advantages and Disadvantages of Liposomes
Advantages of Liposomes
The main advantages of liposomes are considered over their use in drug formulation and delivery. Some of its advantages are:
- Liposomes can deliver both water and lipid-soluble drugs.[5]
- The distinctive structural frames of liposomes allow researchers to create both sustained and targeted drug delivery systems, controlling surface functionalization, size, stiffness, and permeability.[5]
- Liposomes consist of biocompatible ingredients that are perfect for drug distribution as it prevents the oxidation of drugs during their delivery.[5]
- Liposomes are biodegradable, have lower systemic toxicity, improved pharmacokinetic effects, targeted delivery, and sensitive molecules protection.[5]
- Liposomes can reduce serious incompatibilities and side effects originating from systemic drug absorption.[5]
- Liposome increases the efficacy and therapeutic index of drugs, like actinomycin-D.[8]
- Liposomes have increased stability due to encapsulation.
- Liposomes are non-toxic, biocompatible, non-immunogenic for systemic and non-systemic administrations.[8]
- Liposomes can reduce the toxicity of encapsulated agents, like amphotericin B and taxol.[8]
- Liposomes avoid the exposure of sensitive tissues to toxic drugs.
- Liposomes aggregate poorly in soft tissues like the kidney and heart. Thus, entrapped drugs, such as anti-cancer therapies, anti-inflammatory drugs, and anti-infection drugs, don’t cause any damage to the tissues. This effect is called the site avoidance effect.[10]
- Liposomes are flexible to couple with site-specific ligands; thus, they are considered better for achieving active targets.
- Liposomes can be used to target specific cells or tissues.[1]
- Liposomes can carry large fragments of DNA—even as large as chromosomes.[1]
Disadvantages of Liposomes
Despite having all the advantages above, liposomes have some limitations that prevent their extensive uses in clinical and therapeutic areas. These include:
- Low solubility in aqueous solutions.[5]
- Short half-life in the body environment.
- High production cost.
- Due to the large sizes of liposomes, several challenges are involved in using them for tissue targetings, such as leakage and fusion of the loaded drugs, allergic reactions to some liposomal compounds, and phospholipids oxidation and hydrolysis.[5]
Applications of Liposomes
- Site-specific drug delivery: Liposomes are suitable vehicles for drug delivery at targeted locations. To form a site-specific drug delivery system, they are combined with opsonins and ligands, such as antibodies, sugar residues, apoproteins, or hormones, which are tagged on the lipid vesicles.[10] This also helps in reducing drug-related toxicity.
- Cancer therapeutics: Liposome-based cancer therapeutics are used to treat breast cancer in a site-specific manner. It enhances the pharmacokinetics and pharmacodynamics of the associated drug and delivers it to its intended site, increasing its therapeutic efficiency.[10]
For example, Anthracyclines are anticancer drugs. However, they kill the tumor cells and inhibit the other fast-dividing cells of the body, including hair, blood cells, and gastrointestinal mucosa. This toxicity to other cells can be prevented by using liposomes. Liposomes encapsulate the drugs and accumulate only at the tumor site, increasing drug efficiency and reducing toxicity.[10]
- Transdermal drug delivery: The main challenge in transdermal drug delivery is the penetration of macromolecules and hydrophilic drugs through the stratum corneum (outer layer of the skin).[10] Liposomes have a similar molecular composition as lipid layers and have high permeability. Thus, they are a suitable carrier for the delivery of drugs penetrating the skin.
- Treatment of parasitic diseases and infections: Liposomes serve as an ideal carrier to deliver the drugs to treat parasitic diseases, especially those that infect monocytes and macrophages cells, like leishmania.
- HIV (human immunodeficiency virus) infection treatment: Liposomes can encapsulate anti-HIV nanocarriers, like antiretroviral nucleotide and other antiviral drugs, and deliver them to specific sites.[10]
- Disease diagnosis: Liposomes are used for therapeutic imaging modalities, such as X-ray and nuclear magnetic resonance imaging.
- Cosmetics: Liposomes act as a perfect carrier for the delivery of many dermatological products to skin cells. They are used in formulations that avoid dry skin, thus preventing skin aging, and act as a delivery vehicle for enhancers, anti-inflammatory agents, and immunostimulants.[10] It prevents age spots, dark circles, wrinkles, and acne.
Broadly, liposomes’ advantages in cosmetology are:[10]
- Improved penetration and diffusion of active ingredients in cosmetic products.
- Selective transport of active ingredients.
- Greater stability of active ingredients.
- Reduced side-effects.
- High biocompatibility.
- Agriculture industry: Liposomes can entrap unstable compounds such as antimicrobials, flavors, antioxidants, and bioactive elements and protect them from a variety of environmental and chemical changes, such as enzymatic chemical changes, ionic strength variations, and temperature change. Thus, they are used to develop new flavors, control flavor release, improve food color, and modify the texture of food components.[10] It also ensures the release of the ingredients at the desired time.
- Immune Response: Liposomes are used to encapsulate antigens for passive and active immunization and to create antibodies. Moreover, they are also used as an immunological adjuvant for allergens, hepatitis B-derived polypeptides, adenovirus type 5 hexon, subunit antigens from the influenza virus, and polysaccharide-protein conjugates.[10]
- Deliver genetic materials: Liposomes are used to deliver genetic materials, like DNA fragments, to targeted cells to synthesize specific proteins.
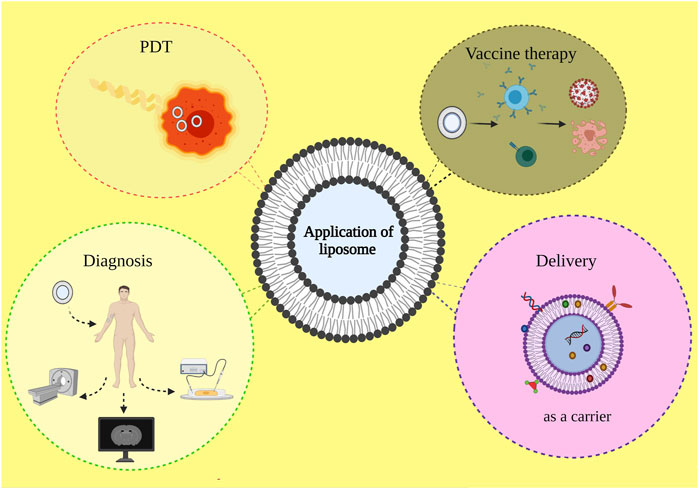
Figure: An illustration of different application areas of liposomes: photodynamic therapy, drug delivery, disease diagnosis, and vaccine therapy.[5]
Conclusion
Liposomes are small spherical vesicles consisting of different phospholipids, cholesterol, and other molecules. Their structural organizations make them perfect as carriers for transporting and delivering a wide range of drugs.
Thus, they have extensive applications in diverse areas, including disease diagnosis, drug and gene delivery, contrast agents for medical imaging, and model cell membrane. They offer several advantages over other available therapeutics, including site-specific-drug delivery, reduced toxicity, biocompatibility, improved efficiency, and many more.
Besides having multiple advantages and their use in several medical areas, liposomes are not without limitations. However, scientists worldwide are working to reduce these limitations, leading to their extensive use in diagnostic assays, immunotherapy, and targeted delivery of drugs.
Thus, emerging scientists hold a golden opportunity to unravel the potential of liposomes in clinical therapeutic applications, leading to better options of diagnosis and treatments for fatal diseases.
References
- Daraee, H., Etemadi, A., Kouhi, M., Alimirzalu, S., & Akbarzadeh, A. (2014). Application of liposomes in medicine and drug delivery. Artificial Cells, Nanomedicine, and Biotechnology, 44(1), 381–391. doi:10.3109/21691401.2014.953633
- Ahmed, K. S., Hussein, S. A., Ali, A. H., Korma, S. A., Lipeng, Q., & Jinghua, C. (2018). Liposome: composition, characterization, preparation, and recent innovation in clinical applications. Journal of Drug Targeting, 1–58. doi:10.1080/1061186x.2018.1527337.
- Liposomes. Retrieved from https://www.precisionnanosystems.com/workflows/formulations/liposomes.
- Composition and Structure of Liposomes. Retrieved from https://www.creative-biolabs.com/lipid-based-delivery/composition-and-structure-of-liposomes.htm.
- Nakhaei P, Margiana R, Bokov DO, Abdelbasset WK, Jadidi Kouhbanani MA, Varma RS, Marofi F, Jarahian M, and Beheshtkhoo N (2021) Liposomes: Structure, Biomedical Applications, and Stability Parameters With Emphasis on Cholesterol. Front. Bioeng. Biotechnol. 9:705886. doi: 10.3389/fbioe.2021.705886.
- Nisini R, Poerio N, Mariotti S, De Santis F and Fraziano M (2018) The Multirole of Liposomes in Therapy and Prevention of Infectious Diseases. Front. Immunol. 9:155. doi: 10.3389/fimmu.2018.00155.
- Walde, P., Beterelli Giuliano, C., Cvjetan, N., & Ayache, J. (2020). Multivesicular Vesicles.− Preparations and Applications. ChemSystemsChem. doi:10.1002/syst.202000049.
- Akbarzadeh, A., Rezaei-Sadabady, R., Davaran, S., Joo, S. W., Zarghami, N., Hanifehpour, Y., Samiei, M., Kouhi, M., & Nejati-Koshki, K. (2013). Liposome: classification, preparation, and applications. Nanoscale research letters, 8(1), 102. https://doi.org/10.1186/1556-276X-8-102.
- Liposomes – properties and bursting. Retrieved from https://www.wikilectures.eu/w/Liposomes_-_properties_and_bursting.
- Karami, Nasim & Moghimipour, Eskandar & Salimi, Anayatollah. (2018). Liposomes as a Novel Drug Delivery System: Fundamental and Pharmaceutical Application DEFINITION AND HISTORY. 12. 10.22377/ajp.v12i01.2037.