Introduction: More Than a Startle—Jumping as a Behavioral Signal
Jumping in rodents is one of the most striking and kinetically intense behaviors observed in the laboratory. It appears spontaneously in open-field tests, elevated plus mazes, novel object paradigms, and during escape or exploratory routines. While often dismissed as mere overactivity or startle response, jumping is a multifaceted behavior—rooted in evolutionary survival, shaped by environmental context, and regulated by complex neural circuits. At Conduct Science, we recognize that understanding these vertical movements can unlock new perspectives on rodent cognition, affective state, and sensorimotor integration.
Jumping may emerge in a variety of experimental and naturalistic contexts: from exploratory leaps in search of escape, to anxious bouncing in confined chambers, to structured, rhythmic hopping in enriched environments. Each variation carries a distinct set of neurobehavioral implications. Far from being an erratic or disruptive action, jumping is a communicative and diagnostic behavior, one that reflects the interplay between motivation, perception, and neurological control.
Neuroethological Basis of Jumping in Rodents
Jumping behavior in rodents is more than a sudden leap—it is an evolutionarily conserved, neurologically coordinated survival mechanism. As a part of the rodent’s natural behavioral repertoire, jumping serves functions ranging from predator evasion to environmental navigation and social signaling. Unpacking the neuroethological underpinnings of this behavior reveals a remarkable interplay between sensory perception, motor execution, emotional valence, and environmental context. In this way, jumping is not just a biomechanical act, but a lens through which researchers can study the neural logic of behavior.
In wild settings, jumping enhances a rodent’s ability to escape quickly from predators, traverse uneven terrain, and reach otherwise inaccessible spaces—like elevated ledges or food sources. This form of vertical locomotion requires rapid integration of sensory cues with precisely timed motor output. Before the leap, a rodent typically pauses, scans the environment, and gathers visual, olfactory, and tactile information. This preparatory phase involves the superior colliculus, which integrates visual signals, and the somatosensory cortex, which processes tactile feedback from the environment.
The actual execution of the jump is controlled by descending motor pathways, particularly those involving the basal ganglia, cerebellum, red nucleus, and spinal cord motor neurons. The basal ganglia play a critical role in initiating the motor program and ensuring that the jump is executed in a goal-directed manner. Meanwhile, the cerebellum refines the movement, coordinating posture, balance, and trajectory. This ensures that the rodent not only launches with sufficient force but also lands safely—a process that requires continuous sensorimotor feedback and fine-tuned timing.
Importantly, jumping is not purely reflexive. It is modulated by internal state and contextual learning. For instance, rodents learn through experience which surfaces are jumpable, where threats are most likely to emerge, and how high or far they must leap to clear obstacles. These decisions are influenced by prefrontal cortical regions, especially in complex or novel environments, indicating a cognitive component to jumping. As such, impaired jumping in rodent models of prefrontal cortex dysfunction—such as in schizophrenia or autism models—can reflect deficits in executive processing, risk assessment, or environmental mapping.
In pathophysiological contexts, alterations in jumping behavior may serve as early signs of motor system pathology. For example, rodents with cerebellar lesions or spinocerebellar ataxias exhibit poor coordination during jumps, often stumbling during takeoff or missing the intended landing zone. Similarly, rodents with dopamine depletion (as seen in Parkinson’s models) may be unable to initiate jumping due to bradykinesia or rigidity. In contrast, hyperdopaminergic states, such as those induced by amphetamine sensitization or certain genetic mutations (e.g., in DAT or DRD2), often produce excessive or repetitive jumping, indicative of heightened arousal or compulsive motor output.
Interestingly, jumping may also be entrained or modulated by environmental predictability. In chronically enriched cages with vertical elements (such as platforms or ramps), rodents learn to incorporate jumping into their daily exploratory routines. In such settings, the behavior can become structured, rhythmic, and even purposeful—distinguishing it from the chaotic, escape-like jumping observed in anxiogenic environments. These differences underscore the importance of context in interpreting jumping behavior and highlight the adaptive flexibility of this locomotor act.
Jumping also appears to be species- and strain-specific. For example, laboratory mice such as C57BL/6J or BTBR T+tf/J strains differ markedly in their propensity to jump in open-field or home-cage settings. While the former may jump infrequently unless startled, the latter is known for engaging in vertical jumping and backflipping—behavior interpreted as repetitive or stereotypic and often used as a marker for autism-like traits in rodent models (McFarlane et al., 2008). These differences are not merely motoric—they reflect underlying variation in neural circuitry, dopaminergic tone, and cognitive-emotional processing.
Moreover, the neural substrates for jumping are developmentally regulated. Young rodents tend to engage in more exploratory jumps as they master spatial navigation and develop muscular strength, while older rodents may exhibit reduced jumping due to age-related muscle loss or neurodegeneration. Measuring changes in jumping behavior across the lifespan can thus offer valuable insights into aging, neuroplasticity, and motor decline.
From a research perspective, jumping provides a quantifiable endpoint for studying complex behaviors with both affective and motor components. At Conduct Science, our mission is to provide researchers with platforms and technologies capable of capturing these fleeting yet informative events. With high-speed video tracking, vertical detection algorithms, and 3D maze environments, we empower scientists to measure jumping behavior with granularity, precision, and contextual depth.
In sum, the neuroethological basis of jumping reflects the remarkable capacity of the rodent brain to produce goal-directed, adaptive motor behaviors in response to environmental challenges. By studying this behavior through a neuroethological lens, researchers can bridge the gap between neural activity, motor output, and ecological function—advancing our understanding of both normal and pathological brain function.
Jumping as a Marker of Emotional Arousal and Anxiety
In the behavioral repertoire of rodents, few actions are as sudden, forceful, and unmistakable as the leap. While jumping is often instinctual and motor-driven, it is also one of the most emotionally loaded behaviors a rodent can exhibit in a controlled environment. When interpreted through the lens of neuroscience, jumping becomes a powerful behavioral marker for emotional arousal, anxiety, panic, and agitation—a window into the rodent’s affective state and the inner dialogue between survival instincts and environmental threat perception.
Rodents in the wild rely on jumping for immediate escape. In the presence of a predator, tight space, or unpredictable threat, vertical escape is an efficient evasive maneuver. This same instinctive reaction manifests in laboratory settings, particularly when rodents experience stress, novelty, or confinement. Within behavioral paradigms such as the Open Field Test, Elevated Plus Maze, or novel cage environment, jumping often emerges during periods of peak anxiety or emotional dysregulation.
When a rodent perceives no viable path of escape, it may resort to vertical motion as a last-ditch effort to flee an overwhelming situation. In such cases, jumping represents not exploratory behavior but panic-driven escape behavior—one that has been likened to flight responses seen in acute stress reactions. This form of jumping is typically accompanied by other high-arousal indicators such as elevated heart rate, increased grooming, defecation, or freezing behavior between leaps.
Importantly, jumping in this context is often context-sensitive. For example, in the Elevated Plus Maze, rodents that jump off the open arms are typically those that exhibit high trait anxiety or low threshold for environmental stressors. Such animals may show extreme avoidance behaviors or choose perceived escape—even at the cost of injury—over continued exposure to aversive conditions. This aligns closely with human panic responses, in which perceived entrapment triggers erratic or harmful escape attempts.
Jumping can also reflect anticipatory anxiety. In the Open Field Test, rodents placed in the center of a brightly lit arena—a situation mimicking predator exposure—may begin jumping near walls or corners, especially if they do not perceive any escape routes. This behavior becomes more pronounced in animals exposed to prior stressors, anxiogenic compounds (e.g., yohimbine, CRF agonists), or genetic predisposition to anxiety-like traits. The frequency and duration of jumping thus become quantifiable metrics of heightened arousal and threat response.
Moreover, pharmacological studies have shown that anxiolytic agents—such as benzodiazepines or SSRIs—reduce the incidence of jumping in stress-inducing environments. This reduction is often dose-dependent and correlates with other behavioral indicators of reduced anxiety, such as increased exploration or time spent in open arms of a maze. As a result, jumping is not only a symptom of heightened arousal but also a behavioral endpoint for drug screening and therapeutic efficacy evaluation.
In some cases, excessive jumping transitions into repetitive, stereotypic behavior, particularly in chronic stress models or neurodevelopmental disorders. In these contexts, jumping loses its clear adaptive function and becomes compulsive or perseverative—as seen in rodent models of autism spectrum disorder (ASD) or obsessive-compulsive disorder (OCD). For example, BTBR T+tf/J mice, a commonly used ASD model, exhibit repetitive jumping and backflipping in novel environments, especially when deprived of environmental enrichment. In such cases, jumping reflects emotional dysregulation combined with motor disinhibition, offering a distinct signature of psychiatric distress.
From a physiological standpoint, jumping behavior under anxiety is mediated by a network of brain regions associated with arousal, threat detection, and motor coordination. The amygdala plays a central role in evaluating threat and triggering defensive responses. Once activated, it recruits downstream circuits in the periaqueductal gray (PAG) and hypothalamus, which mobilize autonomic arousal and prime the motor system for escape. The locus coeruleus, a key source of norepinephrine, amplifies cortical arousal, while the motor cortex, basal ganglia, and cerebellum coordinate the jump itself. This multi-level neuroaxis ensures that emotional arousal is rapidly converted into motor output—a hallmark of adaptive survival behavior.
Jumping, then, is not just an escape. It is a behavioral fingerprint of fear, a muscular echo of internal distress, and a quantifiable marker of emotional imbalance. When rodents jump, they are not merely reacting—they are revealing their perception of threat, their neurochemical state, and the balance (or imbalance) of systems that regulate their engagement with the world. In these vertical bursts lies a wealth of information for neuroscience to decode.
Environmental and Genetic Modulation of Jumping
Jumping behavior in rodents is not merely a spontaneous reaction to a stimulus—it is a context-dependent expression of both environmental conditions and genetic predisposition. While it may appear sudden and instinctive, the frequency, intensity, and style of jumping are shaped by an intricate web of factors, ranging from housing conditions to strain-specific neurobiology. When properly measured, these jumping behaviors serve as a biobehavioral readout for the interaction between genes and environment (GxE)—a foundational principle in behavioral neuroscience.
Environmental Influence: Stress, Enrichment, and Novelty
The environment in which a rodent is housed plays a pivotal role in shaping the expression of jumping behavior. In standard laboratory cages, where stimulation is minimal, rodents may develop what is often referred to as boredom-induced hyperactivity. Under-stimulated animals, particularly in isolation, may exhibit frequent, high-intensity jumping as an outlet for physical and sensory frustration. This behavior often reflects a form of stress-reactive hyperarousal, and when repetitive, can become stereotypic—a symptom of chronic under-enrichment.
In contrast, rodents housed in enriched environments—equipped with running wheels, tunnels, platforms, and toys—display a different pattern of jumping. Here, vertical movements are often exploratory and goal-directed. Mice may leap between platforms, climb structures, or rear and jump in response to novelty. These structured jumping bouts are not signs of distress but rather of positive arousal and engagement, linked to improved synaptic plasticity, hippocampal neurogenesis, and better performance on cognitive tasks (Rosenzweig & Bennett, 1996).
Jumping behavior also responds acutely to situational novelty. In a new testing environment, especially one with limited exit cues—such as an open field arena or clear-walled chamber—rodents may jump in an attempt to escape perceived confinement. This jumping typically occurs after an initial exploratory phase and may intensify over time, indicating a shift from exploratory to escape motivation. Such transitions offer a unique insight into the rodent’s emotional processing and adaptability.
Environmental stressors such as bright lights, loud sounds, or sudden cage changes can also trigger exaggerated jumping, especially in high-reactivity strains. These responses are often measured in models of post-traumatic stress disorder (PTSD) or sensory processing disorders, where environmental triggers can provoke startle-like vertical motion.
Genetic Modulation: Strain Differences and Mutant Models
Genetic background significantly influences jumping behavior, often producing strain-specific behavioral phenotypes. For instance, C57BL/6J mice, one of the most widely used strains in neuroscience, typically show moderate jumping behaviors under stress but are generally tractable and easy to habituate. In contrast, BTBR T+tf/J mice—a commonly used model for autism spectrum disorder—display excessive vertical jumping and backflipping, behaviors interpreted as motor stereotypies and anxiety-linked hyperactivity (McFarlane et al., 2008).
Other strains, such as DBA/2 or FVB/N, may exhibit low baseline jumping but become hyper-responsive to certain drugs or environmental triggers, highlighting the role of dopaminergic sensitivity and neurochemical regulation in jump initiation. These inter-strain differences underscore the importance of choosing appropriate genetic backgrounds when designing studies involving open field, stress response, or motor behavior.
Genetic modifications also offer critical insights. Rodents with dopaminergic dysregulation, such as DAT knockout mice (dopamine transporter-deficient), often display exaggerated jumping and locomotor responses to novelty and psychostimulants. Similarly, mutations in NMDA receptor subunits, often associated with schizophrenia or cognitive disorders, produce hyperactive jumping behavior linked to impaired glutamatergic signaling.
Models of motor neuron diseases or ataxia, such as those with cerebellar Purkinje cell degeneration, may show reduced or uncoordinated jumping, highlighting deficits in motor planning and proprioception. In contrast, hyper-jumping behaviors may arise in genetic models of epilepsy, particularly during pre-ictal arousal or seizure onset.
Interestingly, some transgenic models exhibit circadian modulation of jumping behavior, where gene knockouts related to clock genes (like Per1/Per2 or Bmal1) result in arrhythmic activity bursts, including sudden, non-purposeful jumps. These observations align jumping behavior not only with neuromotor function but also with internal timekeeping systems.
Epigenetic and Developmental Influences
Beyond pure genetics, early-life experiences—such as maternal separation, prenatal stress, or nutritional deficiencies—can epigenetically program jumping tendencies. Rodents exposed to chronic early stress are more likely to exhibit jumping as a startle or panic response, reflecting altered stress axis sensitivity (hypothalamic-pituitary-adrenal or HPA axis).
Developmental factors also modulate jumping. Juvenile rodents often engage in playful jumping, but as they mature, the behavior becomes more context-bound and emotionally driven. In aging rodents, decreased jumping ability can indicate neuromuscular degeneration, loss of muscle mass, or impaired motivation—metrics valuable in aging and sarcopenia research.
Pharmacological Relevance and Measurement Implications
In pharmacological research, jumping is both a side effect and a symptom. Many stimulant drugs—including amphetamines, caffeine, and modafinil—induce dose-dependent increases in jumping behavior in rodents. This hyperlocomotion is often used to assess drug-induced behavioral activation, dopamine reuptake inhibition, or excitotoxicity at high doses. Conversely, drugs that dampen CNS activity—such as benzodiazepines, antipsychotics, and sedatives—often reduce or eliminate jumping, offering a behavioral index of sedation.
Accurately measuring jumping behavior, however, poses unique challenges. Vertical movements can be difficult to detect using standard infrared beam-break systems or horizontal motion-tracking tools. At Conduct Science, we support researchers with 3D video tracking, high-speed cameras, and custom arena designs that allow the vertical plane to be quantified reliably. This enables precise measurement of:
- Jump count
- Jump height and force
- Inter-jump interval
- Jump location and escape targeting
- Stereotypy index (to distinguish functional vs. compulsive jumping)
Such data are essential for interpreting behavioral pharmacology studies and disease models with hyperlocomotive or stereotypic phenotypes.
Jumping in Maze-Based and Cognitive Testing
Jumping behavior also has implications in spatial cognition and decision-making tests, including classic Conduct Science paradigms like the Barnes Maze, Elevated Plus Maze, and Open Field Test. For instance, a mouse that jumps off the edge of a Barnes Maze rather than using available escape holes may not be cognitively impaired—but instead hyper-reactive or poorly habituated to the task environment. In such cases, jumping provides a confounding variable that must be accounted for in latency and error scoring.
Moreover, frequent jumping during cognitive tasks may mask true learning ability. A rodent under high emotional stress may abandon task-solving strategies in favor of escape attempts, leading to artificially poor performance. Measuring jumping alongside traditional task metrics offers a more comprehensive behavioral profile, bridging cognitive outcomes with emotional state.
Conclusion: Capturing the Vertical Dimension of Rodent Behavior
Jumping is far more than a physical motion—it is a behavioral expression of biology meeting environment, a motor act imbued with meaning, shaped by evolutionary survival, emotional state, genetic code, and experiential context. Within a single leap lies a cascade of neural decisions, molecular signals, muscular calculations, and instinctive reflexes. When a rodent jumps, it is not merely displacing its body in space—it is broadcasting its internal reality to the observer.
What makes jumping particularly powerful as a research tool is its multidimensional diagnostic capacity. It reflects stress, sensory processing, arousal, learning, and motivation all at once. Its emergence in barren environments as a cry of under-stimulation, or in enriched cages as a celebration of exploration, paints a striking narrative of how animals adapt—or fail to adapt—to their surroundings. When repetitive and purposeless, it warns us of circuit dysfunction; when precise and goal-driven, it confirms neural harmony.
At Conduct Science, we urge researchers not to view jumping behavior as noise to be filtered, but as data to be interpreted—a vertical signal in a field too often constrained to the horizontal. By pairing high-resolution analytics with customizable environments and sensitive behavioral frameworks, we can harness this underutilized behavior to illuminate the intricacies of neurological function, psychiatric states, and environmental resilience.
As we deepen our understanding of the genetic and environmental determinants of jumping, we also deepen our grasp of how behavior emerges from the living brain—fused with context, charged with history, and ready to leap. And in that leap lies not only a reaction, but a message.
Let us listen.
References
- McFarlane, H. G., Kusek, G. K., Yang, M., Phoenix, J. L., Bolivar, V. J., & Crawley, J. N. (2008). Autism-like behavioral phenotypes in BTBR T+tf/J mice. Genes, Brain and Behavior, 7(2), 152–163. https://doi.org/10.1111/j.1601-183X.2007.00330.x
- Rosenzweig, M. R., & Bennett, E. L. (1996). Psychobiology of plasticity: Effects of training and experience on brain and behavior. Behavioural Brain Research, 78(1), 57–65. https://doi.org/10.1016/0166-4328(95)00216-2
- Thomas, A., Burant, A., Bui, N., Graham, D., Yuva-Paylor, L. A., & Paylor, R. (2009). Marble burying reflects a repetitive and perseverative behavior more than novelty-induced anxiety. Psychopharmacology, 204(2), 361–373. https://doi.org/10.1007/s00213-009-1466-y
- Crawley, J. N. (2008). Behavioral phenotyping strategies for mutant mice. Neuron, 57(6), 809–818. https://doi.org/10.1016/j.neuron.2008.03.001
- McFarlane, H. G., et al. (2008). Autism-like behavioral phenotypes in BTBR T+tf/J mice. Genes, Brain and Behavior, 7(2), 152–163.
- LeDoux, J. E. (2000). Emotion circuits in the brain. Annual Review of Neuroscience, 23(1), 155–184. https://doi.org/10.1146/annurev.neuro.23.1.155
- Thomas, A., et al. (2009). Marble burying reflects a repetitive and perseverative behavior more than novelty-induced anxiety. Psychopharmacology, 204(2), 361–373.
Written by researchers, for researchers — powered by Conduct Science.
See more of Our Posts
Behavioral Neuroscience
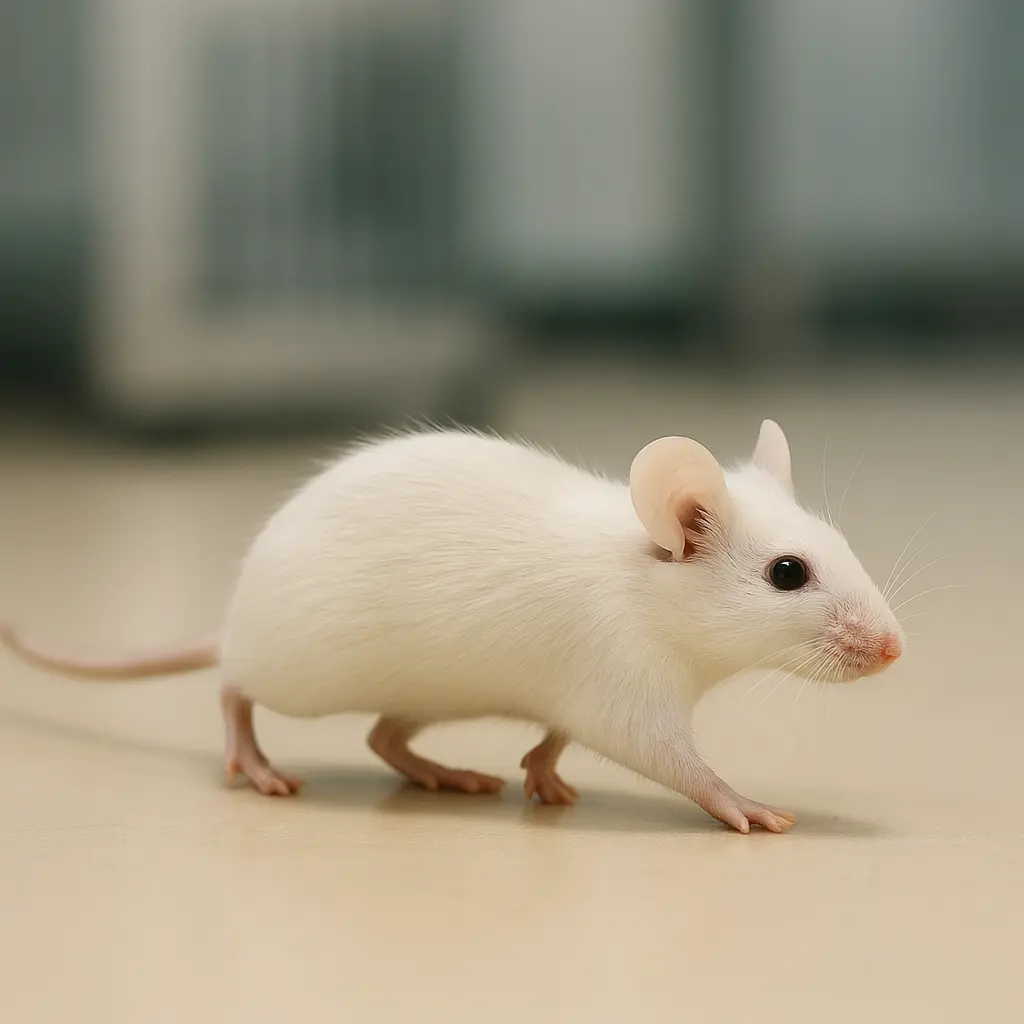
Vertical Vigilance: Exploring Supported and Unsupported Rearing in Rodents

Walking in Rodents: Movement, Gait, and Motor Control

Why Do Rodents Jump? Exploring the Science Behind Their LeapsReferences

Why Rodents Rest: The Science Behind Their Sleep, Nests, and Quiet Moments



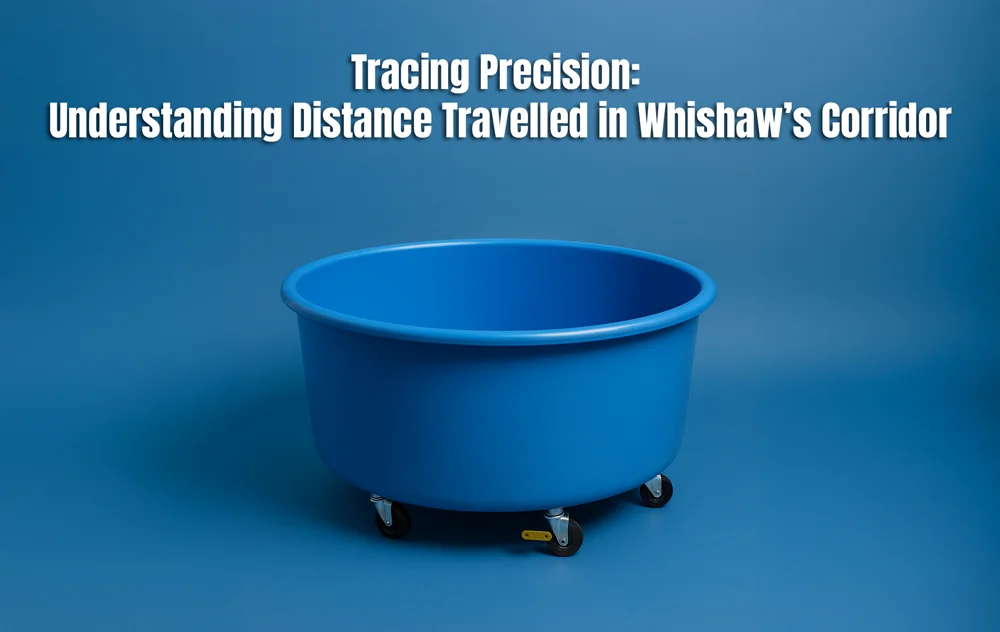
Tracing Precision: Understanding Distance Travelled in Whishaw’s Corridor
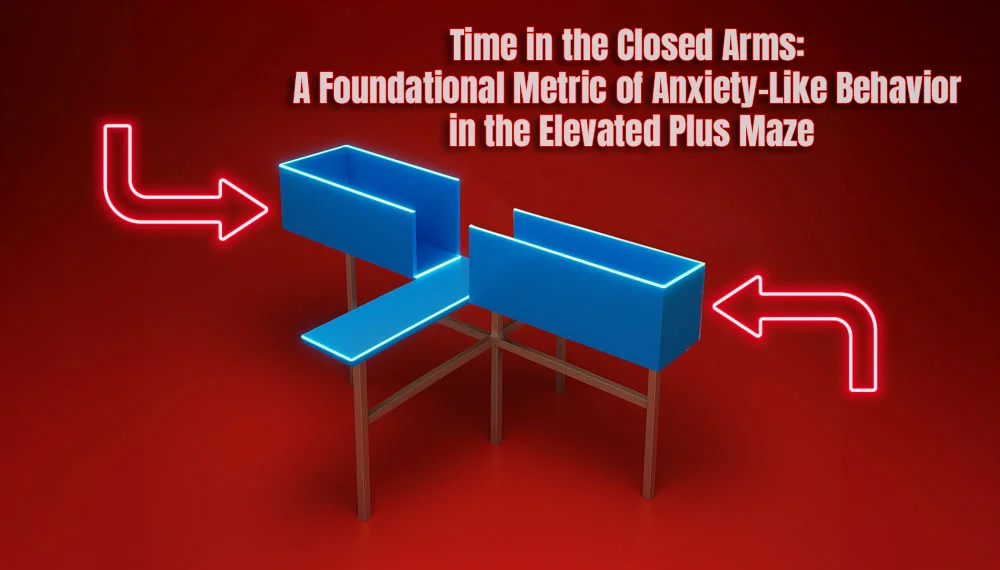
Time in the Closed Arms: A Foundational Metric of Anxiety-Like Behavior in the Elevated Plus Maze
