Description
Mouse Acoustic Startle |
Speaker |
Transducer |
Mouse Grid Rod |
Sound Attenuation Chamber |
Conductor Software; with Startle Reflex; Prepulse inhibition; Fear potentiation startle protocols |
Rat Acoustic Startle |
Speaker |
Transducer |
Mouse Grid Rod |
Sound Attenuation Chamber |
Conductor Software; with Startle Reflex; Prepulse inhibition; Fear potentiation startle protocols |
Features
- Speaker: Raise and lower volume based on intrachamber volume (from the detector)
- Two independent channels, each with Range 100-20,000Hz; 1-120dB
- White Noise Generator included in default Software.
- Specify the duration of time and Hz of white noise
- Easy synchronization for acoustic startle
- Insert any sound file you wish to play using the Conductor Software
- Dual visible and IR light bulb.
- Sound (Hz) Detector
- Speaker Frequency: (100-20,000 Hz)
- Speaker Intensity: 1-120 dB
- The transducer converts the vertical movement of the platform into a voltage signal
- Easy fit with Maze Engineers Animal holders
- Visible and IR light dual bulb
- 0.1-4.0mA in 0.1mA steps
- DC Current.
- Removable Grid
- Optional Airpuff module for shock purposes
- $890
- Custom sound file and set-up available upon request
Grid Rod Animal Holder (Small) For animals weighing 0-50 g
- Interior: LxWxH (cm): 6.04 x 6.19 x 4.80
- Base: LxWxH (cm): 25.4 x 8.89 x 0.318
- Rods Spacing: 0.795 cm apart
- Side Rods overall diameter: 0.318 cm
- Bottom Rods overall diameter: 0.318 cm
Grid Rod Animal Holder (Medium) For animals weighing 125 – 250 g
- Interior: LxWxH (cm): 16.5 x 6.09 x 5.08
- Base: LxWxH (cm): 25.4 x 8.89 x 0.318
- Rods Spacing: 0.978 cm apart
- Side Rods overall diameter: 0.318 cm
- Bottom Rods overall diameter: 0.47 cm
Grid Rod Animal Holder (Large) For animals weighing 250 – 500 g
- Interior: LxWxH (cm): 16.5 x 8.1 x 9.1
- Base: LxWxH (cm): 25.4 x 8.89 x 0.318
- Rods Spacing: 1.4 cm apart
- Side Rods overall diameter: 0.318 cm
- Bottom Rods overall diameter: 0.47 cm
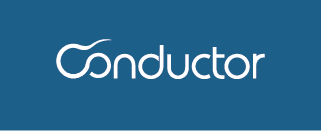
Take advantage of Neuralynx, Ethovision Integration, SMS and Email integration with the Conductor Science Software. No I/O Boxes Required

Introduction
The Acoustic Startle Response Chamber is employed to study the mechanisms underlying sensorimotor integration. This chamber measures the startle response, a defensive reflex triggered by abrupt or threatening auditory stimuli, often resulting in rapid eye closure and muscle contractions. In animals, this response can occur in situations resembling predator encounters and is therefore also termed the escape response (Fetcho & McLean, 2009). The startle circuitry involves short neural pathways with fast-conducting neurons responsive to various sensory inputs, which then connect to large motor neurons that exhibit strong excitation when exposed to startle stimuli (Fetcho & McLean, 2009). This response is valuable for exploring cellular functions and synaptic connections.
Although reflexive in nature, the startle response can also be influenced by other sensory stimuli, such as sensory gating (Fendt, Li, & Yeomans, 2001). Deficits in sensory gating, assessed through pre-pulse inhibition, are often associated with neuropsychiatric disorders like schizophrenia (Mena et al., 2016) and obsessive-compulsive disorder (Ahmari, Risbrough, Geyer, & Simpson, 2016). Such impairments are also seen in neurological conditions such as Huntington’s disease (Swerdlow et al., 1995) and Tourette syndrome (Zebardast et al., 2013). Consequently, the acoustic startle response is instrumental in evaluating sensory gating deficits and developing related treatments. Additionally, it can be used to investigate learning and emotional responses.
The Acoustic Startle Response Chamber features a sound-proof isolation chamber, an animal holder with a grid floor, and a transducer. Stimulus delivery is managed through speakers, a visible light bulb, and a shock grid attachment. The chamber can be calibrated with Conductor Software. Other devices used for reflex and behavior assessments include the Step Down Avoidance, Geotaxis Test, Electrical von Frey Filament, and Predator Odor Exposure Test.
Apparatus and Equipment
The chamber allows for the presentation of various stimuli, including auditory, electrical, and visual. Auditory stimuli are delivered through two independent speakers, each with a frequency range from 100 to 40,000 Hz and an intensity range of 1 to 150 dB. A sound detector is integrated to help manage volume levels. Visual cues are generated using both visible light and infrared bulbs. For electrical stimuli, a removable DC shock grid is available, with the shock intensity adjustable in 0.1 mA increments ranging from 0.1 to 4.0 mA.
Training Protocol
The protocol is structured into three main phases: acclimation, habituation, and pre-pulse inhibition. Throughout the session, a constant white background noise should be maintained.
Ensure that subjects are accustomed to handling for a minimum of one week before starting the sessions. If a subject has undergone surgery, provide at least one week of recovery time to avoid any surgical stress affecting the performance.
Allow the subject to acclimate to the testing room for at least 30 minutes without any stimuli. Before placing the subject in the apparatus, weigh it and then let it adjust to the apparatus with the background noise (white noise at 65 to 68 dB) activated for 5 to 15 minutes.
Following the acclimation phase, begin the habituation phase using only startle stimuli. Present the subject with 30 to 100 startle stimuli in the range of 105 to 120 dB having a duration between 20 to 40 msec. Though debated, it is recommended that the intertrial interval between the startle stimuli be randomized between 10 to 30 sec to prevent the possible prediction of the timing of the stimuli by the subject. However, fixed intervals may also be used.
Allow the subject 5 to 10 minutes acclimation period before beginning the pre-pulse inhibition phase. Begin the session with 4 to 5 successive bursts of startle pulse (120 dB for 40 msec) to normalize the subject response. Follow this trial with a pseudorandomized presentation of startle pulse, no stimulation and three distinct pre-pulse (for example 70, 75, and 80 dB for 20 msec) followed by startle pulse (after 30 or 100 msec). Perform at least 10 trials under each of the three conditions with inter-trials interval randomized between 10 to 30 sec (a fixed interval of 20 sec may also be used). A pre-pulse alone condition may also be included in the pre-pulse inhibition block.
The startle threshold trial allows the establishment of the appropriate startle pulse and is usually performed following the habituation phase. Present each decibel in a randomized order for a 40 msec duration. Present at least 3 to 5 instances of each decibel and average it.
For long term habituation protocol, the general protocol (acclimation phase, habituation phase, and pre-pulse inhibition phase) are to be repeated over a course of at least 5 consequent days. It must be ensured that all conditions, including housing and timing of the experiment, are maintained across all days with very little variability.
The long-term habituation assessment can also be performed only with the acclimation phase and the habituation phase, with the habituation phase having at least 100 startle pulse used.
Literature Review
Wah, Ossenkopp, Bishnoi, and Kavaliers (2018) investigated the effects of repeated adolescent stress as well as propionic acid on the behaviors and the response to sensory stimuli of Long-Evans rats. Based on the drug; sodium propionate (PPA, 500 mg/kg) and phosphate-buffered saline (PBS) male subjects (post-natal day 27) were divided into 4 treatment groups: cat-odor PPA, cat-odor PBS, no odor PPA and no odor PBS. The subjects were exposed to cat odors obtained from worn collars (male and female cats) for 30 minutes across 2 weeks (post-natal days 28, 30, 32, and 34) in a Predator Odor Exposure Test chamber. Controls were exposed to the odor of unworn collars in the same set-up. On post-natal day 40, the subjects were intraperitoneally administered the treatment drugs at 2.0 ml/kg body weight. Fifteen minutes following the administration, behavioral alterations were assessed in the Light-Dark Box. On post-natal day 43, subjects were once again treated with the drugs, 10 minutes following which they were evaluated in the Acoustic Startle Response Chamber (acoustic startle response, 120 dB; pre-pulse inhibition, 76 and 82 dB). Treatment procedures and behavioral assays were repeated once again on post-natal day 74 (Light-Dark Box) and 77 (Acoustic Startle Response Chamber) to evaluate the changes in adulthood behaviors. While no significant changes in the duration of time spent in the light chamber were found between the cat-odor exposed groups, the groups did show decreased nose pokes into the light area compared to their controls. In comparison to PBS treated groups, PPA treatment decreased the number of transitions between compartments, decreased corrected total distance traveled, and decreased corrected vertical time in both adolescents and adults. No effect on the magnitude of the response could be observed between the cat odor groups, or the PPA treated groups. Pre-pulse inhibition testing at 76 dB revealed a decreased percentage of pre-pulse inhibition in PPA treated groups in comparison to the PBS groups. However, no significant differences between groups were observed at 82 dB.
Story, Chan, Munro, Rossignol, and Dunbar (2018) observed that the 5xFAD mice model displayed similar startle response as Alzheimer’s disease sufferers. Twelve 5xFAD mice and 9 wild types consisting of both male and females (8 months in age) were evaluated for their startle reflex in the Acoustic Startle Response Chamber. Acclimation was carried out twice a day for 3 to 5 days using 65 dB white noise for 5-minute intervals. Following this period subjects received 30 pseudorandomized trials that included 10 startle trials (120 dB, 50 msec) and 20 (10 + 10 trials) pre-pulse trials. Pre-pulse (30 msec) was generated as an 8 or 16 dB increase in white noise which was followed 70 msec later by 120 dB startle pulse. The average inter-trial interval was approximately 24 seconds, and the startle response was recorded in the 250 msec window following the startle pulse. In comparison to their wild type counterparts, 5xFAD mice displayed a significant reduction in peak startle response and a significant increase in the latency to peak startle response (mean latency 83.9 msec). Pre-pulse inhibition trials revealed that the 5xFAD maintained the baseline startle response while the wild types displayed significantly lower pre-pulse inhibition at both pre-pulse increments (70% at 8 dB increment and 51% at 16 dB increment). Subjects were also tested in the Open-Field. However, no significant differences in performances could be observed.
Subjects were offspring of Wistar rats that had received a single intravenous injection of either poly I:C (4 mg/kg) or saline on day 15 of gestation. The subjects (aged more than 90 post-natal days) underwent surgery for placement of electrodes in the mPFC (ventral prelimbic cortex; anteroposterior (AP) +3.5, mediolateral (ML) 0.6, dorsoventral (DV) -3.4) or the Nacc shell. Following surgery, the subjects were allowed to recover for 1 to 2 weeks and received habituation to handling, deep brain stimulation procedure as well as the test chamber during the last 5 days. The pre-pulse inhibition trials were carried out in three sessions: session 1 served as the baseline performance and did not involve deep brain stimulations, session 2 included deep brain stimulation of the mPFC or the Nacc, and session 3 was used to evaluate if session 2 effects continued and thus did not include brain stimulation. The sessions were spaced 24 hours apart. Subjects were placed in the Acoustic Startle Response Chamber and underwent acclimation (5 min) with 60 dB background noise and 10 startle stimuli (20 msec) following which test session commenced. Test session trials included 10 presentation of each 1 pulse alone (100dB, 20 msec), 1 no stimulus, 2 pre-pulse alone (72 dB or 68 dB, pure tone, 10 Hz, 20 msec), 3 pre-pulse (72 dB, 68 dB, or 64 dB) followed by pulse after 100 msec trials. A significant decrease in the pre-pulse inhibition was observed in the poly I:C offspring group, relative to saline offspring group, in sessions 1 and 3, which was normalized by deep brain stimulation (both mPFC and Nacc group) in session 2. On the other hand, Nacc deep brain stimulation-induced pre-pulse inhibition deficits in the saline-treated dams’ offspring. (Bikovsky et al., 2016)
Halberstadt, Slepak, Hyun, Buell, and Powell (2016) investigated the “k” analog, methoxetamine, for causing behavioral changes similar to PCP and “k”. Male Sprague-Dawley rats were housed in 12-hour reverse light/dark cycle to allow testing in the awake phase. Subjects were administered 1 ml/kg injections of either “p” hydrochloride (PCP) (0.8, 1, or 1.2 mg/kg); (±)-N-allylnormetazocine hydrochloride (NANM) (2.5, 5, or 10 mg/kg); S-(+)-“k” hydrochloride (1.5, 3, 6, or 12 ,g/kg); R-(−)-“k” hydrochloride (1.5, 3, 6, or 12 ,g/kg); and methoxetamine hydrochloride (MXE) (1, 3, or 10 mg/kg). The effects of each drug were evaluated in the Acoustic Startle Response Chamber 15 min after treatment with NANM, 5 min after treatment with MXE or PCP, and immediately after treatment with “k”. Startle sessions included pseudorandomized trials of 12 pulse-alone (120 dB, 40 msec) and 36 pre-pulse (12 trials each at 68, 71, and 77 dB) + pulse trials. Additionally, subjects were also presented with 6 pulse alone trials before and after testing. At doses 3 and 10 mg/kg of MXE, resulted in a decrease of pre-pulse inhibition at all 3 pre-pulse intensities. Startle response observation revealed a 3-fold increase in the magnitude of response at a dose of 1 mg/kg. While PCP did not affect the amplitude of the response, it resulted in a significant decrease in pre-pulse inhibition at all intensities. Similarly, “k” isomers (S-(+)-“k” hydrochloride and R-(−)-“k” hydrochloride) did not have any effect on the magnitude of the response. Though both resulted in reduced pre-pulse inhibition, in comparison to the R-(−)-“k”, S-(+)-“k” proved to be more potent. Further, only S-(+)-“k” showed a drug and pre-pulse intensity interaction with no reduction in the pre-pulse intensity at 68 dB. NANM reduced pre-pulse inhibition with 10 mg/kg reducing pre-pulse inhibition at all intensities while 5 mg/kg showing a significant reduction only at 68 and 71 dB. NANM also did not affect the startle amplitude. Overall, MXE was observed to have resulted in behavioral alterations similar to PCP.
Data Analysis
In addition to the observation of behavioral reactions, the following data can be recorded in the Acoustic Startle Inhibition Chamber,
- Peak startle response amplitudes
- Average startle response amplitudes
- Percentage of pre-pulse inhibition
%PPI = [pulse-alone – (pre-pulse + pulse score)]/pulse-alone score
- Reflex latency
Strengths and Limitations
Behavioral outcomes of subjects can be influenced by fluctuations in lighting and noise levels within the holding area due to housing conditions. Variations in the isolation chamber’s environment, such as changes in background noise between trials, can lead to inaccurate data. Additionally, improper calibration of the apparatus components may compromise data quality. Residual olfactory or other cues from previous trials might also affect performance. Factors such as the subject’s age, gender, weight, species, and sensitivity to the stimuli can further impact their performance.
Summary
- The Acoustic Startle Response Chamber is used in the assessment of sensorimotor gating, learning, and emotions.
- The sound-attenuated chamber minimizes the influence of external auditory stimuli on the performances.
- The chamber accommodates multiple animal holders, thus allowing simultaneous assessments in groups of animals.
- The Acoustic Startle Response Chamber can be used for other protocols such as fear-potentiated startle, emotions assessment, learning assessment, stress induction, and hearing assessment.
References
- Ahmari, S. E., Risbrough, V. B., Geyer, M. A., & Simpson, H. B. (2016). Prepulse Inhibition Deficits in Obsessive-Compulsive Disorder are More Pronounced in Females. Neuropsychopharmacology, 41(13), 2963–2964. doi:10.1038/npp.2015.363
- Bikovsky, L., Hadar, R., Soto-Montenegro, M. L., Klein, J., Weiner, I., Desco, M., … Hamani, C. (2016). Deep brain stimulation improves behavior and modulates neural circuits in a rodent model of schizophrenia. Experimental Neurology, 283, 142–150. doi:10.1016/j.expneurol.2016.06.012
- Curzon, P., Zhang, M., Radek, R.J., & Fox, G.B. (2009). The Behavioral Assessment of Sensorimotor Processes in the Mouse: Acoustic Startle, Sensory Gating, Locomotor Activity, Rotarod, and Beam Walking. In Buccafusco, J. J., & Buccafusco, J. J. Methods of behavioral analysis in neuroscience. Boca Raton: CRC Press.
- Fendt, M., Li, L., & Yeomans, J.S. (2001). Brain stem circuits mediating prepulse inhibition of the startle reflex. Psychopharmacology, 156(2-3):216-24.
- Fetcho, J. R., & McLean, D. L. (2009). Startle Response. Encyclopedia of Neuroscience, 375–379. doi:10.1016/b978-008045046-9.01973-2
- Halberstadt, A. L., Slepak, N., Hyun, J., Buell, M. R., & Powell, S. B. (2016). The novel “k” analog “m” produces dissociative-like behavioral effects in rodents. Psychopharmacology, 233(7), 1215–1225. doi:10.1007/s00213-016-4203-3
- Mena, A., Ruiz-Salas, J. C., Puentes, A., Dorado, I., Ruiz-Veguilla, M., & De la Casa, L. G. (2016). Reduced Prepulse Inhibition as a Biomarker of Schizophrenia. Frontiers in Behavioral Neuroscience, doi:10.3389/fnbeh.2016.00202
- Story, D., Chan, E., Munro, N., Rossignol, J., & Dunbar, G. L. (2018). Latency to startle is reduced in the 5xFAD mouse model of Alzheimer’s disease. Behavioural Brain Research. doi:10.1016/j.bbr.2018.07.021
- Swerdlow, N. R. (2009). Prepulse Inhibition of Startle in Humans and Laboratory Models. Encyclopedia of Neuroscience, 947–955. doi:10.1016/b978-008045046-9.01938-0
- Swerdlow, N. R., Paulsen, J., Braff, D. L., Butters, N., Geyer, M. A., & Swenson, M. R. (1995). Impaired prepulse inhibition of acoustic and tactile startle response in patients with Huntington’s disease. Journal of Neurology, Neurosurgery & Psychiatry, 58(2), 192–200. doi:10.1136/jnnp.58.2.192
- Valsamis, B., & Schmid, S. (2011). Habituation and Prepulse Inhibition of Acoustic Startle in Rodents. Journal of Visualized Experiments, (55). doi:10.3791/3446
- Wah, D. T. O., Ossenkopp, K.-P., Bishnoi, I., & Kavaliers, M. (2018). Predator odor exposure in early adolescence influences the effects of the bacterial product, propionic acid, on anxiety, sensorimotor gating, and acoustic startle response in male rats in later adolescence and adulthood. Physiology & Behavior. doi:10.1016/j.physbeh.2018.11.003
- Yeomans, J. S., & Frankland, P. W. (1995). The acoustic startle reflex: neurons and connections. Brain Research Reviews, 21(3), 301–314. doi:10.1016/0165-0173(96)00004-5
- Zebardast, N., Crowley, M. J., Bloch, M. H., Mayes, L. C., Wyk, B. V., Leckman, J. F., … Swain, J. E. (2013). Brain mechanisms for prepulse inhibition in adults with Tourette syndrome: Initial findings. Psychiatry Research: Neuroimaging, 214(1), 33–41. doi:10.1016/j.pscychresns.2013.05.009